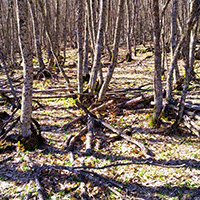
Effects of silvicultural thinning on stand structure and coarse woody debris in the deciduous Arasbaran forest, Iran
iForest - Biogeosciences and Forestry, Volume 16, Issue 6, Pages 377-384 (2023)
doi: https://doi.org/10.3832/ifor4312-016
Published: Dec 19, 2023 - Copyright © 2023 SISEF
Research Articles
Abstract
Forest stand structure is influenced by artificial factors such as silvicultural activities, and by natural factors such as wildfires, floods, windstorms, diseases, and insect infestations. The silvicultural treatments used during the transformation of coppice to the coppice-with-standard system are a main source of coarse woody debris (CWD). Even small changes in the amounts and/or types of CWD can threaten forest biodiversity, including plant and bird diversity. In this study, we compared managed and unmanaged forest stands in Northwest Iran to better understand changes in species composition and stand characteristics (with particular reference to CWD) in relation to silvicultural practices. In total, thirty-six 0.5-hectare sampling plots were surveyed (total area: 18 ha). We found significant differences between the cut (active management) and uncut areas (proforestation) in indices of height, number of tree species, canopy cover of live and fallen trees, dead tree density, and relative frequency of dead trees. The sample plots included 22 species of live trees, and the CWD was generated by 16 of these species. The number of stems of live trees in the sample plots was 2.653 (SE = 181), with Carpinus orientalis accounting for the highest mean density of live trees. C. orientalis also provided the highest total frequency of downed logs (70%) in the stands, followed by Quercus macranthera (20%). Q. macranthera accounted for the highest relative frequency of dead trees in the stands. In total, around 42% of the CWD consisted of logs lying on the forest floor, followed by dead trees (39%) and stumps (19%). Most of the CWD was included in decay class (DC) 2 (71.6%), followed by DC1 (16.7%) and DC3 (11.3%). Forest managers must balance the amount of CWD, especially from dead trees, in these stands to conserve the diversity of the material in terms of both decay class and tree species.
Keywords
Coppice-with-standard System, Quercus macranthera, Silvicultural Activities, Structural Characteristics, Tree Removal
Introduction
Forests play a crucial role in carbon dioxide removal (CDR), which is imperative for meeting the temperature targets set by the Paris Climate Agreement in 2015. These targets aim to limit global warming to either 1.5 or 2.0 °C ([18]). Maximizing the carbon sequestration potential of existing forests is a key strategy for enhancing CDR, mitigating climate change impacts, preserving biodiversity, and safeguarding the quality of air, land, and water resources. Natural forests, in particular, are identified as the most effective carbon reservoirs ([16]).
In contrast, managed plantations, subject to periodic harvesting, have significantly lower carbon storage capacity due to the continuous maintenance of young, small trees. Additionally, plantations often practice monoculture, resulting in slower carbon sequestration rates compared to undisturbed forests with greater tree species diversity and higher biological carbon sequestration potential ([17]). Recent research in tropical regions reveals that natural forests contain an astonishing 40 times more carbon than plantation forests ([16]).
Alternative approaches to carbon removal in forest ecosystems include afforestation (establishing new forests) and reforestation (replacing forests on previously deforested or recently harvested lands). These strategies are crucial for addressing both global climate change and biodiversity loss. One noteworthy approach within this context is “proforestation”, which involves allowing previously managed areas to naturally develop, thereby promoting continuous growth, carbon accumulation, and structural complexity in existing natural forests. Proforestation can complement other forest-based solutions such as afforestation, reforestation, and improved forest management practices. However, it is important to note that while afforestation and reforestation contribute to CDR, newly established forests typically take several decades to a century before they can sequester substantial amounts of carbon dioxide ([18]).
Numerous studies in many countries have shown that reforestation can provide habitats for a wide range of native forest plants, animals and fungi and can support a diverse array of native understorey plants ([20], [4]). Reforestation programmes are widely implemented in many parts of the world to create microhabitats for different types of fauna ([19]). Nevertheless, the use of afforestation to enhance biodiversity remains controversial, as it may actually have the opposite effect. Poorly managed afforestation that does not consider plant diversity may cause an increase in invasive species and a reduction in native populations ([22], [4]).
Coarse woody debris (CWD) is considered a main factor supporting forest biodiversity ([36]). CWD generally consists of dead and downed wood such as dead trees, logs, stumps and logging residues ([31], [27]). It also includes large dead branches and large pieces of wood lying on the forest floor. CWD is typically generated by artificial treatments and by natural factors such as wildfires, floods, windstorms, diseases and insect infestations ([27]). CWD is also produced naturally by the death and decomposition of trees and is dependent on site productivity; in managed forests, CWD can even be produced as a result of silvicultural activities ([29], [13]). Human involvement sometimes intervenes to modify the expected distribution of CWD components. CWD provides a habitat for insects, cavity-nesting birds and small and large mammals; the insect populations living within CWD can be a source of food for both mammalian and avian species ([35], [29]). The quantity and quality of CWD are key factors in maintaining, e.g., a high diversity of beetles ([24]). The importance of CWD in forests can be evaluated by, e.g., the quantity influenced by tree mortality, dimension, stem quantity, decay conditions and tree species.
The amount of CWD is not stable and is influenced by natural and human-based drivers, including forest management and timber harvest; human access can also have substantial effects on CWD density ([2], [37]). Research on forest ecosystems has focused on the role of CWD in ecosystem functioning and the effects of artificial and natural disturbance on the amount, condition and dynamics of CWD ([37]). Several studies have examined the amount, quality, distribution and rates of decay of CWD and dead trees in forest ecosystems ([31], [33], [34], [36], [2], [27]). The impact of silviculture treatments on CWD in Southwest mixed-conifer forests (USA) has also been investigated by Saud et al. ([29]), who emphasized some silvicultural prescriptions, including prescribed fire, as a useful tool for altering CWD to meet management objectives. In another study, the impact on CWD of human access to forests has been studied in the Hyrcanian forests in Iran ([2]). The effects of timber harvesting and fire history on CWD in the dry sclerophyll jarrah (Eucalyptus marginata) forest of south-western Western Australia have also been examined ([37]). Intensively managed forests are characterized by significantly lower mean levels of deadwood C than yielded by other forms of management ([23]). In the north of Iran, comparison of the number of dead trees in three sites showed that dead tree volume differed significantly in sites with different management history, reaching highest levels in a virgin site and lowest levels in a site with a long-term history of management ([32]).
Different types of management have been applied in the Arasbaran forest (NW Iran) since it became a protected area in 1973, including wood harvesting, wildfire mitigation and, more recently, conservation management. The stands were initially intensively harvested for fuelwood (about 48 years ago) and were then managed as a biosphere reserve, with some areas given special protection for conservation. As part of the conservation plan, silviculture treatment, including thinning, was used in these forests to transform coppice to the coppice-with-standard system. However, this operation has led to the accumulation of CWD and has contributed to changing the forest structure (Fig. 1). To the best of our knowledge, no previous studies have evaluated long-term forest protection programmes regarding the amount and quality of CWD in these forests. The aim of this study was to compare active managed and unmanaged stands (proforestation) in the Arasbaran forest to better understand changes in species composition and stand characteristics (with particular reference to CWD) in relation to silvicultural practices.
Fig. 1 - Silviculture thinning in the managed sites in the Arasbaran forest, NW Iran (photo: S. Ghanbari).
Methods
Study area
This research was conducted in the Arasbaran forest region in Northwest Iran, at the border of Armenia and Azerbaijan, between latitude 38° 35′ N - 39° 00′ N and longitude 45° 45′ E - 47° 05′ E (Fig. 2). The average elevation of the three sites is between 1500 and 2260 m above sea level, respectively. The climate in the area is semi-humid, with an average annual temperature of 14 °C and an average rainfall of 400 mm per year. One of the most important influences on human disturbances is fuelwood harvesting. Natural, mixed hardwood and broad-leaved deciduous forests cover an area of about 153,000 ha. The origin and composition of the stands in the Arasbaran forest are relatively similar, and the stand structure depends on the type of management. The main tree species in the forests are Carpinus betulus and Quercus macranthera, with Acer campestre present as an accompanying species.
Fig. 2 - Location of study area including cut (red points) and uncut (yellow points) sample plots in the Arasbaran forest, NW Iran.
The occupations of the local people in the target watershed are primarily based on combinations of animal husbandry, farming, carpet-weaving, beekeeping, and cultivation or extraction of forest products ([5], [7]). The conservation and management plan of Arasbaran, which is called “Siyanat”, began in 2001 (22 years ago). This plan also was implemented in the Hyrcanian and Zagros forests of Iran. The “Siyanat” conservation plan is aimed at the quantitative and qualitative assessment of forest stands with some silviculture thinning, following the principles of sustainable forest management, as well as monitoring of standing volume, regeneration, species diversity, and stand composition.
Data collection and analysis
In general, the forests in the district are managed as a mixed forest with stands thinned from below. The thinned stands are defined as management sites, but other areas of the Arasbaran forest have been protected since 1973, and no management activities, such as tree removal, have since been carried out in these sites. Thinning intensity is calculated as the percentage of the stand basal area removed relative to the basal area of control plots ([9]). A transformation thinning operation, specifically thinning from below or selective thinning, was carried out in the stands 22 years ago. Furthermore, the most recent management activities, involving the cessation of wood harvesting for wood fuel purposes, took place 48 years ago. The thinning operation exhibited an intensity of approximately 20-25% in terms of the number of trees per hectare, and this thinning operation occurred singularly in 2001. All cut trees remained in the forest stands. Data were collected from May 2020 to September 2022 in forest stands of the study area. In order to determine the effect of forest types on CWD and live trees, the main forest types were identified in the cut and uncut stands. The three main forest types in the cut stands were Quercetum, Carpinetum, and Querco-Carpinetum. In each forest type, we surveyed 6 sample plots in a randomly systematic distribution. The number of plots for each treatment is always the same. The grid (of dimensions 800 × 800 m) intersected at the centre of each sampling plot. In each site, which included the cut (active management) and uncut areas (proforestation), we established 18 sample plots of dimensions 100 × 50 m (0.5 ha). In total, we inventoried 36 sample plots and a total area of 18 ha.
In each plot, we measured the percent slope, slope aspect, elevation, and crown canopy percent (CCP). We then recorded the species, diameter at the breast height (dbh), and height of all live trees of the different species, as well as dbh, height, decay class, and status of dead trees (stump, log, or standing) as CWD. Trees of DBH ≥ 5 cm and height ≥ 1.3 m were considered mature. The stem volumes were calculated by Huber’s formula (V = BAm × h) where BAm is the mid-point cross-sectional area (m2) and h is the height (m). Standing dead trees or snags (dbh ≥ 5 cm - hereafter snags), downed logs (minimum diameter ≥ 5 cm and length ≥1 m), and stumps (defined as the cut tree remains) were examined in each plot as CWD. Species, dbh, height, volume, and decay class were recorded for each sampled snag, downed log, and stump. Based on the field measurements, we calculated 12 characteristics describing the forest stand structure and related to both living and dead trees, including: (1) number of tree species; (2) live tree density (n ha-1); (3) mean DBH (cm); (4) height (m); (5) basal area (m2 ha-1); (6) maximum dbh (cm); (7) canopy cover (%); (8) stumps (n ha-1); (9) fallen trees or logs (n ha-1); (10) snags (n ha-1); (11) dead tree density (n ha-1); and (12) percentage of dead trees ([1]). These characteristics were determined for different forest types and also for all forest types in the cut (active management) and uncut areas (proforestation).
The botanical species of the CWD was assessed from the bark and its sprout group. The dbh and length of the downed logs and stumps were recorded with tree callipers and a metre stick. The height of the snags was measured with a clinometer. The decay class (DC) of the snags, logs and stumps was categorized as follows: (i) DC1 - recently dead trees with intact tops and most fine branching present, structure round, leaves and bark present, cambium still fresh, wood solid, wood colour original, more than 75% of wood still intact and a good part of the bark still intact, with twigs and leaves attached; (ii) DC2 - 25-50% of wood beginning to soften, and bark only partially converted, lacking fine twigs and leaves; (iii) DC3 - 75% of the tree trunk has decayed, and bark totally degraded; (iv) DC4 - trees with broken tops and few or no coarse branches, heartwood decayed soft, leaves absent, bark often absent, wood colour original to faded, all of the stems on the ground, more than 75% of wood has decayed ([30], [36], [27]).
Species basal area, density, and relative importance value (RIV) were calculated in each plot for both live and dead trees. The RIV was calculated for each species by averaging the relative density (number of stems) and the relative dominance (basal area) values. RIV ranks tree species within a site on the basis of the total number of individual trees and also the total amount of forest area occupied by the tree species. This index indicates how frequently a species occurs across the entire forest. The RIV of the CWD was calculated for each species in each plot by averaging the sum of each relative density and relative volume of CWD ([26], [33]). Finally, the three most important species, Q. macranthera, C. orientalis, and Acer campestre, were considered in terms of RIV.
The relationships between basal area (volume of CWD), density and RIV values for trees and CWD and plot parameters (slope aspect, slope, elevation and canopy cover) were investigated using Pearson’s product-moment correlation coefficients. For slope aspect categories as nominal data sets, the non-parametric Spearman’s rank correlation procedure was used instead of Pearson’s product-moment correlation coefficients. The effects of cutting as a management factor and forest type as a fixed factor were evaluated by ANOVA with dependent variables for live stands including the number of tree species, live tree density, mean DBH, height, basal area, and maximum dbh. Dependent variables for dead trees were stumps, logs, snags, and dead tree density per ha, and percentage of dead trees. The t-test analysis was employed to compare the means of two groups of forest types in the cut (active management) and uncut areas (proforestation). Statistical tests were calculated using R v. 3.5.3 ([25]) and Microsoft Excel™ v. 2013 depending on the type of analysis. A significance level of α ≤ 0.05 was applied in all statistical tests.
Results
Live trees and amounts of CWD
A total of 22 species of live trees were identified in the sample plots, which demonstrates the high level of tree species diversity in this forest ecosystem and its enormous importance for biodiversity conservation.
In total, 2653 ± 181 (SE) stems per ha of live trees were found in the sampling plots, with C. orientalis present at the highest mean density. For the other variables, Q. macranthera yielded the highest value, followed by C. orientalis and A. campestre (Tab. 1). The density of CWD was 391 ± 154 stem per ha. Three major tree species generated most of the CWD in the sampling plots. The most frequent CWD species were C. orientalis, accounting for 52% of the density in the sample plots, followed by Q. macranthera (35%), and A. campestre (10%). Among all CWD species, Q. macranthera and C. orientalis had respectively the highest and second highest relative density, relative basal area and RIV (Tab. 1).
Tab. 1 - Mean (± standard error) density, basal area, relative density (RELDEN), relative basal area (RELBA) and relative importance value (RIV) of live trees and CWD in the Arasbaran forest, NW Iran. SE is in the parenthesis.
Main forest species | Density (Stems ha-1) |
Basal area (m2 ha-1) |
RELDEN | RELBA | RIV | |||||
---|---|---|---|---|---|---|---|---|---|---|
Live trees |
CWD | Live trees |
CWD | Live trees |
CWD | Live trees |
CWD | Live trees |
CWD | |
C. orientalis | 999 ± 241 | 203 ± 89 | 10.3 ± 2.4 | 1.8 ± 0.1 | 29.5 ± 6.0 | 28.0 ± 6.0 | 27.5 ± 5.7 | 29.8 ± 6.4 | 28.5 ± 5.8 | 29.2 ± 6.2 |
Q. macrathera | 878 ± 106 | 136 ± 48 | 17.6 ± 3.2 | 0.9 ± 0.4 | 35.9 ± 3.5 | 50.0 ± 4.5 | 55.4 ± 4.4 | 50.4 ± 5.0 | 45.6 ± 3.8 | 50.3 ± 4.6 |
A. campestre | 78 ± 18 | 13 ± 5 | 0.8 ± 0.2 | 0.1 ± 0.0 | 3.6 ± 0.8 | 5.3 ± 1.6 | 3.7 ± 0.9 | 4.8 ± 1.3 | 3.6 ± 0.8 | 5.0 ± 1.4 |
Other species | 698 ± 112 | 39 ± 15 | 3.6 ± 0.9 | 0.2 ± 0.1 | 31.0 ± 4.8 | 16.0 ± 2.4 | 13.4 ± 2.2 | 15.5 ± 2.97 | 22.2 ± 3.5 | 15.6 ± 2.6 |
All species | 2653 ± 181 | 391 ± 154 | 32.2 ± 4.0 | 3.0 ± 1.3 | - | - | - | - | - | - |
We found significant differences between the cut (active management) and uncut areas (proforestation) in some structural characteristics of live trees. Regarding the structural characteristics related to live trees, live tree density, mean DBH, height, basal area, maximum dbh and canopy cover (%) were higher in cut stands, while the number of tree species was higher in uncut stands. The number of tree species, height, and canopy cover differed significantly between cut and uncut stands (Fig. 3).
Fig. 3 - Comparison of structural characteristics of live trees in cut and uncut stands in the Arasbaran forest (NW Iran). (a): Live tree density (n ha-1); (b): mean DBH (cm); (c): number of tree species; (d): basal area (m2 ha-1); (e): height (m); (f): maximum dbh (cm); (g): live and dead tree density (n ha-1).
Management had a significant effect on live tree density (F=29.11, p<0.001), basal area of stand per ha (F=13.61, p<0.001) and maximum dbh (F=4.35, p<0.05). Forest type as a fixed factor had a significant effect on live tree density (F=3.96, p<0.05) and mean height (F=5.62, p<0.001). The interaction between management and forest type had a significant effect on the number of tree species (F=7.86, p<0.01), mean dbh (F=6.86, p<0.01), and basal area (F=4.04, p<0.05 - Tab. 2).
Tab. 2 - Results of ANOVA with some dependent variables of living trees and forest type and management as fixed factors.
Source | Variable | F | p-value |
---|---|---|---|
Management | Number of tree species | 0.10 | 0.750 |
Live tree density | 29.11 | <0.001 | |
Mean DBH | 0.35 | 0.561 | |
Mean Height | 3.61 | 0.067 | |
Basal area | 13.61 | <0.001 | |
Max dbh | 4.35 | 0.046 | |
Forest type | Number of tree species | 1.79 | 0.184 |
Living tree density | 3.96 | 0.030 | |
Mean DBH | 1.88 | 0.170 | |
Mean Height | 5.62 | 0.008 | |
Basal area | 2.22 | 0.126 | |
Max dbh | 1.51 | 0.237 | |
Forest type × Management |
Number of tree species | 7.86 | 0.002 |
Living tree density | 1.58 | 0.222 | |
Mean DBH | 6.86 | 0.004 | |
Mean Height | 0.30 | 0.740 | |
Basal area | 4.04 | 0.028 | |
Max dbh | 3.12 | 0.059 |
Correlation analysis revealed that crown cover was positively and significantly related to density (r=0.54, p<0.01) and basal area (r=0.413, p<0.05) of live trees. The slope aspect was negatively and significantly related to the basal area of CWD (r=-0.37, p<0.05). Other variables related to the live trees and CWD were not significantly related to slope, crown cover, elevation or aspect (Tab. 3).
Tab. 3 - Correlation results for density and basal area of living trees and CWD with the topographical variables. (*): p<0.05; (**): p<0.01.
Variable | Density of live trees |
Basal area of live trees |
Density of CWD |
Basal area of CWD |
---|---|---|---|---|
Slope | -0.236 | 0.07 | -0.063 | -0.056 |
Crown cover | 0.540** | 0.413* | 0.253 | 0.234 |
Elevation | -0.288 | -0.266 | -0.286 | -0.282 |
Aspect | -0.019 | -0.078 | -0.274 | -0.37* |
CWD status and decay classes
We found differences between the cut (active management) and uncut areas (proforestation) in some structural characteristics for dead trees (Tab. 4, Fig. 4). Management had a significant effect on log density per ha (F=21.98, p<0.001) and dead tree density per ha (F=13.77, p<0.001). Forest type as a fixed factor had a significant effect on log density per ha (F=5.70, p<0.01) and the percentage of dead trees (F=9.12, p<0.001). The interaction between management and forest type had a significant effect on stump density per ha (F=5.16, p<0.05) and log density per ha (F=4.40, p<0.05 - Tab. 4).
Tab. 4 - Results of ANOVA with some dependent variables of CWD and forest type and management as fixed factors).
Source | Variable | F | p-value |
---|---|---|---|
Management | Stump density per ha | 0.41 | 0.527 |
Log density per ha | 21.98 | <0.001 | |
Snag density per ha | 2.92 | 0.098 | |
Dead tree density per ha | 13.77 | <0.001 | |
Percentage of dead tree | 1.89 | 0.179 | |
Forest type | Stump density per ha | 1.33 | 0.279 |
Log density per ha | 5.70 | 0.008 | |
Snag density per ha | 1.44 | 0.253 | |
Dead tree density per ha | 0.88 | 0.426 | |
Percentage of dead tree | 9.12 | <0.001 | |
Forest type × Management |
Stump density per ha | 5.16 | 0.012 |
Log density per ha | 4.40 | 0.021 | |
Snag density per ha | 2.29 | 0.119 | |
Dead tree density per ha | 0.07 | 0.932 | |
Percentage of dead tree | 0.07 | 0.928 |
Fig. 4 - Comparison of structural characteristics of dead trees in cut and uncut stands in the Arasbaran forest (NW Iran). (a): Percentage of dead trees; (b): Stumps (n ha-1); (c): Snags (n ha-1); (d): Dead tree density (n ha-1); (e): fallen trees or logs (n ha-1).
C. orientalis accounted for 70% of the total frequency of downed logs in the stands, followed by Q. macranthera (20%). In the CWD consisting of snags, Q. macranthera accounted for the highest frequency relative to the other species (Fig. 5). Downed logs accounted for about 42% of CWD, followed by snags (39%) and stumps (19%). Most of the CWD was included in DC 2 (71.6%) followed by DC1 (16.7%) and DC3 (11.3%). Only 0.4% of CWD was included in DC4. The same trend was also observed for the volume percent of the CWD in the decay class, with the highest percentage in DC2 (78.5%), followed by DC1 (13%) and DC3 (8.4%).
Discussion
Management programmes including silvicultural and conservation activities impact the quantity and quality of live trees and CWD ([13]). Conservation activities were first implemented in the Arasbaran forest more than 48 years ago and silvicultural prescriptions, around 20 years ago. Management affects the characteristics of CWD in terms of CWD volume, the volume of different types of CWD, including downed logs, snags, stumps and decay classes (DC).
The present research revealed 22 species of live trees and 16 species of CWD in the forest stands under study, indicating that more intensive management maximized species richness. Similarly, Haeussler et al. ([10]) found that species richness 5 to 8 years after clear-cut logging was 30% to 35% higher than in the old forests. They also stated that silvicultural treatments increase species richness in the managed stands ([10], [24]). Q. macranthera yielded the highest relative importance value, followed by C. orientalis, and these species plus Acer campestre were three major types of live trees and CWD in the forest stands ([10], [24]). Q. macranthera yielded the highest relative importance value, followed by C. orientalis, and these species plus A. campestre were three major types of live trees and CWD in the forest stands. These species have been reported to be the three most important species for fuelwood use by rural people of Iran due to high relative frequency and suitable burning qualities ([6]), and they have also been considered the dominant species in the Arasbaran forest ([28]). The practice of selective species harvesting may result in changes in the species composition. Preference is shown for Pinus wallichiana as fuelwood, because it easily catches fire ([21]), and for Quercus spp., due to its burning and heating quality ([6], [21]). Selective harvesting of C. orientalis for fuelwood may be occurring in unmanaged stands, although other ecological and physiographic, and topographic factors can determine species composition. Further research is needed to clarify this issue. However, Tavankar et al. ([36]) found that CWD composition was similar in harvested and control sites in the Hyrcanian forest (Northern Iran).
About 42% of CWD had downed logs status, followed by snags (39%) and stumps (19%). Our findings showed that downed logs are more abundant than snags, as reported in other studies ([14], [11]). In considering the benefits for nature conservation, Herrero et al. ([11]) suggested that snags should account for at least 50% of CWD volume in managed forests, as they provide critical habitat for many species of cavity-nesting birds ([35], [29]). The relatively lower ratio of snags found in both the managed and unmanaged stands under study may reflect the transitory nature of this component ([11]). The number of snags will increase temporarily after a period of increased tree mortality, but the dead trees will eventually fall over and become downed logs. To promote biodiversity, the proportion, size, and longevity of snags should be taken into consideration in developing forest management guidelines ([12], [11]).
Regarding downed logs, the largest volumes were included in DC2 and DC1. The lower abundance and volume of CWD in DC4 may be related to stand age and timing of silviculture activities in these forests. The present findings are consistent with those reported by Stephens & Moghaddas ([35]), who suggested that the overall lower abundance of CWD in DC4 and DC5 may be the result of harvesting activities in the treatment units over the past 100 years. Kapusta et al. ([13]) also reported that silviculture operations in older stands are intentionally conducted at a lower intensity, which may mean less frequent damage to aged CWD. However, Shorohova & Kapitsa ([34]) found that most of the CWD was included in DC1, while in the present study area, most of the CWD was included in DC2. In managed boreal forests in northern Sweden, Gibb et al. ([8]) reported that the abundance of CWD volume in different decay classes was low and that there may be a consistent removal of CWD through sanitary operations or fuelwood harvesting.
Forest managers have several useful tools for selecting tree species, including different types of regeneration cutting, weed control, protection of trees vulnerable to browsing, and thinning of tree species capable of outcompeting all others ([3]). Low amounts of CWD can threaten forest biodiversity, including plant and bird species diversity. Forest managers must therefore balance the amount of CWD, especially snags, in these stands. Management should aim to conserve the diversity of CWD in terms of both decay classes and tree species ([15]).
Conclusion
Stand properties, including numbers of live and dead trees, are affected by both artificial and natural factors. Forest type and intensity of silvicultural thinning also have different impacts on the stand. Regarding the structural characteristics of live trees, DBH, height, and basal area were higher in cut stands than in uncut stands. In the stands under study in the Arasbaran forest, most of the CWD was generated by C. betulus (43.3%) and Q. macranthera (39.9%). Higher values of the structural characteristics indicated that these stands tend to be sustainable. Altering the stand structure through these elements strengthens sustainable forest management plans. In addition, the structural characteristics of CWD, the main source of nesting and food habitats, vary depending on forest types and management intensity.
References
Online | Gscholar
Gscholar
Authors’ Info
Authors’ Affiliation
Omid Fathizadeh 0000-0002-0696-7090
Dept. of Forestry, University of Tabriz, Tabriz (Iran)
Department of Organisms and Systems Biology, Polytechnic School of Mieres, University of Oviedo, Mieres (Spain)
Corresponding author
Paper Info
Citation
Ghanbari S, Sefidi K, Álvarez-Álvarez P, Fathizadeh O, Abbasnezhad Alchin A (2023). Effects of silvicultural thinning on stand structure and coarse woody debris in the deciduous Arasbaran forest, Iran. iForest 16: 377-384. - doi: 10.3832/ifor4312-016
Academic Editor
Giorgio Alberti
Paper history
Received: Jan 19, 2023
Accepted: Oct 15, 2023
First online: Dec 19, 2023
Publication Date: Dec 31, 2023
Publication Time: 2.17 months
Copyright Information
© SISEF - The Italian Society of Silviculture and Forest Ecology 2023
Open Access
This article is distributed under the terms of the Creative Commons Attribution-Non Commercial 4.0 International (https://creativecommons.org/licenses/by-nc/4.0/), which permits unrestricted use, distribution, and reproduction in any medium, provided you give appropriate credit to the original author(s) and the source, provide a link to the Creative Commons license, and indicate if changes were made.
Web Metrics
Breakdown by View Type
Article Usage
Total Article Views: 132
(from publication date up to now)
Breakdown by View Type
HTML Page Views: 0
Abstract Page Views: 0
PDF Downloads: 83
Citation/Reference Downloads: 0
XML Downloads: 49
Web Metrics
Days since publication: 221
Overall contacts: 132
Avg. contacts per week: 4.18
Article Citations
Article citations are based on data periodically collected from the Clarivate Web of Science web site
(last update: Nov 2020)
(No citations were found up to date. Please come back later)
Publication Metrics
by Dimensions ©
Articles citing this article
List of the papers citing this article based on CrossRef Cited-by.
Related Contents
iForest Similar Articles
Review Papers
Opportunities for coppice management at the landscape level: the Italian experience
vol. 9, pp. 775-782 (online: 04 August 2016)
Research Articles
Effects of traditional coppice practices and microsite conditions on tree health in a European beech forest at its southernmost range
vol. 9, pp. 673-681 (online: 12 March 2016)
Research Articles
Tree-oriented silviculture: a new approach for coppice stands
vol. 9, pp. 791-800 (online: 04 August 2016)
Research Articles
Predicted occurrence of ancient coppice woodlands in the Czech Republic
vol. 10, pp. 788-795 (online: 16 September 2017)
Review Papers
Structure and management of beech (Fagus sylvatica L.) forests in Italy
vol. 2, pp. 105-113 (online: 10 June 2009)
Research Articles
Shrub facilitation of Quercus ilex and Quercus pubescens regeneration in a wooded pasture in central Sardinia (Italy)
vol. 3, pp. 16-22 (online: 22 January 2010)
Research Articles
Oak often needs to be promoted in mixed beech-oak stands - the structural processes behind competition and silvicultural management in mixed stands of European beech and sessile oak
vol. 13, pp. 80-88 (online: 01 March 2020)
Research Articles
Arthropod diversity in pure oak forests of coppice origin in northern Thrace (Turkey)
vol. 8, pp. 615-623 (online: 17 December 2014)
Research Articles
Nitrogen removal and its determinants in hybrid Populus clones for bioenergy plantations after two biennial rotations in two temperate sites in northern Italy
vol. 8, pp. 668-676 (online: 02 February 2015)
Research Articles
Effects of traditional forest management on carbon storage in a Mediterranean holm oak (Quercus ilex L.) coppice
vol. 11, pp. 344-351 (online: 18 April 2018)
iForest Database Search
Google Scholar Search
Citing Articles
Search By Author
Search By Keywords
PubMed Search
Search By Author
Search By Keyword