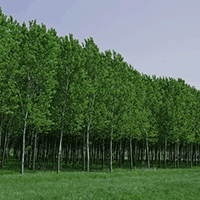
Effective woody biomass estimation in poplar short-rotation coppices - Populus nigra × P. maximowiczii
iForest - Biogeosciences and Forestry, Volume 16, Issue 4, Pages 202-209 (2023)
doi: https://doi.org/10.3832/ifor4200-016
Published: Jul 25, 2023 - Copyright © 2023 SISEF
Research Articles
Abstract
Knowledge of the quantity of woody biomass of poplar short-rotation coppice (SRC) on agricultural land is a basic tool for management decisions like rotation length, volume production and the financial balance sheet of economic activities. The expansion of SRC requires a fast, reliable, easily applicable and cheap method for estimating the biomass yield, but existing methods are based on labour-demanding and lengthy measurements of all shoots per tree. The objective of this study was to verify a novel rapid biomass estimation method that uses averaged attributes of only a few largest shoots as a predictor variable for woody biomass in a poplar SRC, hybrid clone J-105 (Populus nigra × P. maximowiczii). Using data from 39 sample stumps with 187 shoots in total, we modelled shoot biomass as a function of an increasing number of shoots in interaction with different shoot parameters at two poplar SRC plantations. Results showed that the DBH of only three of the largest shoots per stump proved to be accurate estimators of the total shoot biomass of the individual stump. Comparison of biomass estimates at the stand level with a real amount of biomass indicated differences between 6-14%, depending on the site.
Keywords
Poplar Hybrid Clone J-105, SRC, Allometric Equations, Biomass Estimation
Introduction
Growing trees on agricultural land in innovative production systems like a short-rotation coppice (SRC) or agroforestry systems has been recommended by many experts as one of the most promising methods for not only meeting production goals, but also tackling the main problems of current intensive agriculture and consequences of climate change-soil erosion and biodiversity loss ([21], [20], [12], [10]). SRCs utilize the ability of selected fast-growing tree species, usually poplars and willows, to grow quickly and use their high regenerative coppicing ability to create new shoots after repeated harvesting of above-ground organs (trunks, branches). Interest in sprouting stands lies mainly in the fact that they produce on average twice the volume of that of a generative regeneration stand in the first eight years ([37]). The main principles of SRC plantations (hereafter referred to as plantation) were developed in Northern Ireland, England, and Sweden during the 1970s and 1980s as an alternative way of farming, with products for the energy sector and environmental goals such as diversification of agriculture, reduction of greenhouse gases and development of renewables ([15]). In recent years, the use of woody biomass as a renewable raw material has gradually expanded (construction, furniture, horticulture) and we can expect that the diversification trend will continue, especially in the development of the bioeconomy in the EU.
The main advantage of SRCs is that they combine sustainable production of renewable biomass with multiple ecosystem services, such as erosion control, increased biodiversity, carbon sequestration and local cooling. The organic cover (leaf fall) in the young plantation (third-to-fourth year) also has a positive effect on the invertebrate community ([45], [32]). The higher content of organic material (humus) in the soil also significantly reduces water erosion in SRC stands, compared to fields of annual crops ([14]). Poplars have been shown to possess the main useful traits for phytoremediation, such as the ability to grow in nutrient-poor soils, a deep and wide-spreading root system, metal tolerance and a fast rate of growth ([36], [2], [8]).
Non-destructive methods of biomass yield prediction are important either for preparing the appropriate logistic chains for harvest or deciding the date of harvest. Well managed logistics saves time and money. Allometric models with easily measurable tree variables are the most commonly used tool for the estimation of total above-ground biomass in SRCs ([18], [43], [24]). However, common biomass inventory methods are based on measuring all shoots in multi-stem coppiced trees, which is laborious, time-consuming, and therefore costly. In SRCs, only the first rotation is formed by single-stem trees. From the second rotation onwards, numerous shoots usually grow from each stump. This complicates total yield biomass prediction ([29]). That is why we tried to use and verify a simple and rapid method to calculate the amount of woody biomass in plantations of poplar hybrid clone J-105 (also referred to as Max-4). This clone, which has gradually spread into many countries of the temperate zone in Europe ([39]), is cultivated on more than 70% of plantations in the Czech Republic ([44]), and was therefore used in our study.
Clone J-105 is a hybrid between the European black poplar and the East Asian Japanese poplar (Populus nigra L. × P. maximowiczii A. Henry) showing good and stable yields, excellent coppicing ability and high resistance to harmful organisms (roe-buck, Melampsora sp., Chrysomela populi). However, Matula et al. ([17]) and Šrámek et al. ([38]) found a strong allometric relationship between the total woody biomass of all shoots and the averaged parameters of only the three-to-five largest shoots in coppiced trees in traditional coppices. This allometric relationship could also be potentially used for biomass estimation in short-rotation poplar coppices. Nevertheless, it is unclear whether such allometric relationships are applicable to multi-stem fast-growing poplars and which parameter is the best biomass predictor.
To tackle this issue, we aimed (i) to verify the accuracy of the estimation of woody biomass based on allometric equations using the averaged attributes of a few largest shoots as a predictor variable at the SRC plantations, and (ii) to find the combination of the most accurately predicting shoot parameter and the lowest number of shoots on which such a parameter needs to be measured for biomass estimation.
Material and methods
Study site
The study was performed at two existing SRC plantations in the Czech Republic (Tab. 1) where poplar hybrid clone J-105 (Populus nigra L. × Populus maximowiczii A. Henry) was planted. The first site is located near the village of Sobechleby, where the mean annual rainfall of 885 mm and the mean annual temperature of 7.4 °C were recorded from 1981 to 2010 and 9.0 °C in 2020. The second site is located near the village of Vícenice, where the mean annual rainfall of 834 mm and the mean annual temperature of 7.2 °C were recorded from 1981 till 2010 and 8.7 °C in 2020. The names of these villages denote the plantations. The total area of both SRC plantations was 26.2 ha. The SRC plantations were established on agricultural land. According to the Food and Agriculture Organization (FAO) soil classification, Sobechleby is luvisol and Vícenice is kambisol, where the SRC plantations were in the fourth and third year of the second rotation, respectively (Tab. 1). Sobechleby was planted in 2013 and Vícenice in 2015. Both plantations were established in rows 2.5 m apart and 0.5 m between the stumps. Weed control was carried out by disk harrowing during the first year of the first rotation in midsummer. The description of the structural parameters of the SRC plantations and shoots is shown in Tab. 2. Plant mortality ranged 10-14%. In January 2020, Sobechleby was harvested (with the direct-chip harvesting machine), chipped and transported to the heating plant where it was weighed. The same logistics chain was applied to Vícenice in January 2021.
Tab. 1 - Main characteristics of the two poplar SRC sites analyzed in this study.
Characteristics | SRC Site | |
---|---|---|
Sobechleby | Vícenice | |
Area (ha) | 17 | 11.5 |
Clone | J-105 | J-105 |
Age (yrs) | 4 | 3 |
Rotation | 2 | 2 |
Planting density (stump ha-1) | 7692 | 6540 |
Coordinates | 49°28′ 00.139″ N 17°38′ 50.755″ E |
49°05′ 13.164″ N 15°47′ 58.714″ E |
Altitude (m a.s.l.) | 300 | 459 |
Tab. 2 - Poplar short-rotation coppice structure. (BA): stand basal area; (WM): wood moisture.
Variable | SRC Site | |
---|---|---|
Sobechleby | Vícenice | |
DBH (cm) | 3.4 ± 2.1 | 2.8 ± 2.3 |
Height(m) | 5.7 ± 3.1 | 4.9 ± 2.5 |
BA (m2 ha-1) | 14.7 | 14.4 |
No. of stumps (pcs ha-1) | 7.692 | 6.540 |
No. of stems per stump (pcs) | 4.6 ± 0.8 | 4.5 ± 1.1 |
No. of shoots (pcs ha-1) | 35.383 | 29.430 |
WM (%) | 55.9 | 55.5 |
Measurements and sampling
Nineteen poplar stumps with shoots (hereinafter referred to as sample trees) with 98 shoots were selected and destructively sampled at Sobechleby in January 2020 and 20 sample trees with 89 shoots at Vícenice in January 2021. The number of sample trees with shoots was chosen based on the data published in other studies dealing with biomass estimation related to SRC of poplar, i.e., in the range of 10-80 shoots ([1], [43], [25]). The sample tree selection was carried out proportionally to the shoot diameter frequency distribution at each study site. Measurements and sampling were based on methodologies published by Matula et al. ([17]) for measuring biomass in the early post-resprouting period in the lifetime of woody plants and by Šrámek et al. ([38]) for measuring coppice biomass in the post-resprouting period of Quercus cerris L. For every selected sample tree, each individual shoot of the stump was cut and its length (L), diameter 5 cm above its base (BD) and the diameter at breast height (DBH) were measured. The diameters were measured with calibrated ABS SOMET® digital callipers (SOMET CZ, Ltd., Bílina, Czech Republic) with an accuracy of ± 0.1 cm. Then, the sample trees were divided into two components (stems and branches). The fresh weight of all sample tree components was measured directly in the field using the OCS-L® hand portable electronic weight scale, with an accuracy of 0.5 kg (Precision Scale, Calgary, Canada).
All results were analysed and presented as fresh mass of the dendromass (hereafter referred to as biomass).
We measured the stand structure (Tab. 2) on inventory plots in order to estimate the amount of biomass using Field-Map technology and the software pack Coppice II (IFER Ltd. Jílové u Prahy, Czech Republic) on both plantations. We measured 299 inventory plots at Sobechleby and 132 at Vícenice. These plots were generated using stratified random selection and each was represented by ten stumps-with-shoots in a row. The DBH of each shoot on each stump was measured. Plant mortality was recorded according to the number of dead stumps.
Inventory of plantations and sampling of sample trees took place on one day in the week when the plantations were harvested. The wood chips were taken to the heating plant within 14 days after harvest. This short logistics chain was chosen to minimize the variability of changes in the moisture content of the shoots and wood chips.
Samples of wood were taken from ten sample trees to determine the moisture content at both plantations. The wood samples (stem disk about 2 cm thick) were taken from the base, the middle and top parts of the dominant shoots of these trees. The sample tree selection was carried out proportionally to the shoot diameter frequency distribution at both plantations. The wood samples were weighed in the field with the P 221 pocket digital scale, with an accuracy of 0.01 g (Sounon, Wanjiang, China). All wood samples were labelled, transported in paper bags to the laboratory and dried at 80°C until reaching a constant weight and then weighed. Subsequently, the water content of the wood was calculated and expressed as a percentage.
To determine the change in moisture in the wood chips during storage at the depot (where the wood chips are stored until they are taken to the customer), 2 samples of wood chips were taken on the 7th and 14th day of deposition of the wood chips at the depot. Mixed samples of wood chips were taken and weighed on-site.
Data analysis
For each sample tree, the shoots were categorized from the smallest to the largest (thickest) by DBH for the measured shoot parameter (BD, DBH and L). We recorded the value of each parameter of the largest shoot, and calculated the average value of the corresponding parameters of the two largest shoots, then of the three largest shoots, and so forth, until we averaged the values of the parameters of the five largest shoots (hereafter referred to as Lavg, DBHavg and BDavg).
The averaged parameter values (Lavg, in m; DBHavg, in cm; Bdavg, in cm) were used as independent variables in non-linear models (i.e., allometric equations) that we created to predict the total fresh mass of shoot biomass per stump (Biomassstump, kg). The exponential and power-law functional forms were used to fit the data. The absolute growth rate is constant for linear models and increases monotonically for exponential and power-law models. The exponential models are frequently used to analyse growth data and may be suitable in the initial growth stage ([23], [26]). However, power-law models are often used as more flexible non-linear models ([26]). Non-linear growth models are flexible enough to account for varying growth rates ([26]). Recently, ecology has focused its attention on power-law functions for their ability to predict relationships among many aspects of growth ([46]). The formulas used for the two models were (eqn. 1, eqn. 2):
where y is the response variable (Biomassstump), x is the average value of the given parameter (Lavg, DBHavg and BDavg) for the given number of the largest shoots (1-5) on a stump, while a and b are the model coefficients. For each model, we calculated the second-order Akaike Information Criterion (AIC - [3]), the Root Mean Squared Error (RMSE) and pseudo-R2, based on the likelihood-ratio ([16]), with Nagelkerke’s adjustment (pseudo-R2adj - [22]). Based on the calculated differences between the AIC values for exponential and power-law models, we selected those with lower AIC values. In order to calculate shoot biomass estimates of several stumps together, as in the case of the sample trees (Biomassst, kg), plot- or stand-level (biomass ha-1) estimates (hereafter referred to as stand-level biomass estimates - Biomassstand, in t), we simply summed all the Biomassstump and compared these sums with the sums of the weighed shoot biomasses per sample tree (Biomassweighed).
The differences between the total-model biomass and the total-weighed biomass of all sample trees were determined by calculating the relative differences in the data. The total biomass of the SRC per unit area (1 ha) was calculated by the sum of the individual mean fresh mass weights of all stumps of the inventory plots and recalculated to the total biomass of the inventory plots to a unit area of 1 ha. All results were analysed and presented as biomass.
Analyses were performed in R ([41]) using the “nmle” package ([28]) and “qpcR” package ([30]); the diagrams were produced using the “ggplot2” package ([47]).
Results
Biomass estimates of all shoots per tree based on the averaged parameters of the largest shoots
Biomassstump was better estimated by the power-law models than by the exponential models for most of the predictors (Tab. 3) at both sites. All shoot parameters (BD, DBH, L) of the largest shoots were significantly related to Biomassweighed (P < 0.05), but the accuracy of the Biomassstump estimates based on them varied among the number of averaged largest shoots (Fig. 1, Tab. 3). In comparison with BDavg and Lavg, DBHavg was a better predictor due to higher pseudo-R2adj and lower RMSE values (Fig. 1) at both sites. The accuracy of the estimation using DBHavg increased with each shoot added to DBHavg (Fig. 2) up to the three averaged largest shoots, except Vícenice, where pseudo-R2adj was decreasing from the largest to the smallest shoot (Fig. 1). Neither RMSE nor AIC values decreased, nor did pseudo-R2adj values increase after adding the fourth largest averaged shoot in the models with DBHavg alike, as in the models with BDavg and Lavg (Fig. 1). Therefore, the average of the three largest shoots was best in achieving the maximum or near-maximum accuracy for the Biomassstump in Sobechleby. Nevertheless, the prediction based on the DBHavg of only the two largest shoots also had a very high value of pseudo-R2adj = 0.95, slightly lower than when the 3 largest shoots were used (pseudo-R2adj = 0.97). The minimum deviations in accuracy were found after adding the second and the third largest averaged shoots in the models in Vícenice. Adding the fourth and fifth largest averaged shoot in the models did not lead to an increase in the accuracy of predictions.
Tab. 3 - Pseudo-R2adj values and the AIC of the generalized power-law and exponential models for estimation of prediction of the total shoot biomass per stump based on basal diameter (BD), DBH, and shoot length (L) for the given number of the averaged largest shoots (ALS, 1-5). The first value in each cell represents Sobechleby, the second Vícenice.
ALS | Parameter | Power-law model | Exponential model | ||
---|---|---|---|---|---|
AIC | R2 | AIC | R2 | ||
1 | BD | 136.4/118.5 | 0.64/0.94 | 141.2/127.1 | 0.54/0.91 |
DBH | 124.9/119.6 | 0.81/0.94 | 129.7/135.9 | 0.75/0.87 | |
L | 123.1/65.4 | 0.81/0.92 | 125.9/66.9 | 0.79/0.90 | |
2 | BD | 104.9/125.4 | 0.93/0.94 | 110.3/125.5 | 0.91/0.89 |
DBH | 98.1/111.2 | 0.95/0.94 | 107.4/127.2 | 0.92/0.88 | |
L | 113.4/73.9 | 0.89/0.80 | 117.9/72.8 | 0.86/0.82 | |
3 | BD | 100.9/122.6 | 0.94/0.93 | 118.1/122.6 | 0.86/0.83 |
DBH | 91.3/118.7 | 0.97/0.93 | 107.4/139.9 | 0.87/0.77 | |
L | 120.4/67.1 | 0.85/0.67 | 129.8/68.5 | 0.75/0.61 | |
4 | BD | 106.7/78.9 | 0.87/0.94 | 109.9/96.4 | 0.85/0.78 |
DBH | 100.4/83.6 | 0.91/0.92 | 104.2/99.7 | 0.89/0.72 | |
L | 122.1/60.9 | 0.67/0.68 | 122.9/63.9 | 0.68/0.54 | |
5 | BD | 67.7/40.7 | 0.87/0.92 | 68.2/40.7 | 0.86/0.91 |
DBH | 66.9/67.8 | 0.88/0.92 | 67.1/79.6 | 0.88/0.73 | |
L | 79.7/20.1 | 0.62/0.88 | 79.9/20.6 | 0.61/0.86 |
Fig. 1 - The relationship between the pseudo-R2adj and RMSE values of the power-law models for shoot biomass (per stump) estimations and the largest shoots averaged for the given parameter (BDavg, DBHavg or Lavg) used as an estimator in the models. (a, b): Sobechleby; (c, d): Vícenice.
Fig. 2 - Prediction of the sum of the biomasses of all the shoots per stump based on averaged DBHs of 1, 2, 3, 4 and 5 largest shoots per stump. (a): Sobechleby; (b): Vícenice.
The contribution of each individual shoot (Biomassindiv) to the total shoot biomass per stump decreased exponentially, i.e., the largest shoot had the greatest biomass, the second largest shoot had the second greatest biomass, etc. (Fig. 3). For this reason, the majority of the total biomass was concentrated in a few of the largest shoots; for example, more than half of the total shoot biomass per stump was contained in the two largest shoots (89% for both sites), and ≥ 90% of the total shoot biomass was contained in the three largest shoots.
Fig. 3 - Mean relative contribution of the individual largest shoots per stump to the total shoot biomass ranked according to their size per that stump, e.g., 1 indicates the largest shoot, 2 the second largest shoot, etc. The grey zone represents the 95% confidence interval for predictions. (a) Sobechleby; (b) Vícenice.
The predicted total (summed) biomass of Biomassst differed from the total weighed biomass only by ≤ 1.2% in the prediction using BD, and by ≤ 0.06% in the prediction using DBH at both sites (Fig. 4). Likewise, in the prediction using L for the three largest shoots, the accuracy was ≤ 0.5% but only in Sobechleby. The most accurate predictor of the biomass estimates at the stand level was DBH, calculated as an average of the three largest shoots. A sufficient accuracy of ≤ 0.9% was also achieved when using only the largest shoot in predictions and DBH at both sites. The least accurate estimate differed from the real biomass by 58% at Sobechleby and 30% at Vícenice, with an error of 269.6 kg of the total 462.6 kg (Sobechleby) and 76.6 kg of the total 251.7 kg (Vícenice) for the BD parameter from 4 averaged shoots.
Fig. 4 - Relative differences between the total biomass of all sample trees (Biomassst) and total weighed biomass (shown as bars) of all measured shoots in relation to the number of the averaged largest shoots and the relevant parameter used for estimation. For the averaged basal diameter (BDavg) and the diameter at breast height (DBHavg), the 1-5 largest shoots were used and, for the averaged length (Lavg), the 1-5 largest shoots within each stump were used. (a) Sobechleby; (b) Vícenice.
Biomass estimate at the stand level
The biomass at the stand level was estimated by measuring the forest structure on the inventory plots at both plantations and by calculating the wood biomass using the allometric equations. The mean DBH, measured on the three largest shoots was used as a predictor in the allometric equations based on the results above (Tab. 4). The difference between estimated and real biomass was 6.5% at Sobechleby and 14.2% at Vícenice. The biomass estimate using allometric equations with the three largest DBHs (of the shoots of each stump on the inventory plots) averaged was overestimated at Sobechleby and underestimated at Vícenice (Tab. 4).
Tab. 4 - Differences between estimated and real biomass of poplar SRC. (DEAB): deviation of the estimate from the real amount of biomass.
Variable | Parameter | Site | |
---|---|---|---|
Sobechleby | Vícenice | ||
Biomass (t ha-1) |
Estimate for the largest shoots | 130.6 | 140.1 |
Estimate for the 2 largest shoots | 101.9 | 124.1 | |
Estimate for the 3 largest shoots | 89.2 | 113.7 | |
DEAB (%) |
The largest shoots | 36.5 | 33.9 |
The 2 largest shoots | 6.8 | 18.6 | |
The 3 largest shoots | -6.5 | 14.2 |
Discussion
We demonstrated that woody biomass of multi-shoot poplar SRC can be well estimated using the parameters of only one shoot (or two/three when higher accuracy is required), depending on whether the estimation is for the stump or stand level. For sufficiently accurate total biomass estimates, it is enough to measure just the largest shoot for the stump level and the three largest shoots for the stand level. It can be assumed that the number of shoots for the calculation of the total woody biomass will also depend on the number of dominant shoots and the number of dead shoots on the stump.
It is generally concluded that the power-law model estimates biomass better than the exponential model; however, in most studies on short rotation crops, the exponential model is usually applied ([29]).
DBH was a better predictor than BD and L, due to higher pseudo-Radj2 and lower RMSE values. The parameter L from the shoots proved to be least accurate as a biomass predictor. This is likely because the shoots may be broken or their tips killed by frost, fungi, herbivores, of infection; therefore, shoot length may not be representative of the whole shoot ([17]).
The DBH of the three largest shoots is sufficient for nearly perfect estimates with negligible errors (≤ 0.06 %) at the level of all sample trees. Using the parameters of more than the three largest shoots does not lead to more precise estimates and may even make the estimates less accurate for both the stump and stand level. This results correspond with the findings of Matula et al. ([17]), who reported a similar evidence using the same methodology for an early post-resprouting period of five common tree species of the temperate zone (Quercus petraea [Matt.] Liebl., Tilia cordata Mill., Carpinus betulus L., Acer campestre L. and Corylus avellana L.). However, they reported more accurate estimates of shoot biomass using 5 averaged shoots. Findings similar to those obtained in this study were reported for a later post-resprouting period of the traditional Quercus cerris L. coppice ([38]). Mosseler et al. ([18]) also concluded that the average stem diameter of the three largest stems had the strongest relationship to biomass yield compared to measurements based on up to 20 of the largest stems per plant of two native North American willow (Salix) species.
Matula et al. ([17]) stated that most of the shoot biomass in coppiced trees is contained in the 5 largest shoots, which was the best predictor for the biomass of the entire resprouting individual. Šrámek et al. ([38]) found that most of the shoot biomass in the late post-resprouting period in oak coppices is contained in the two largest shoots. In contrast, we found that most of the woody biomass is contained in the 3 largest shoots in our two poplar SRCs. Nevertheless, the correlation between the average size of the few largest shoots and biomass of all shoots was also confirmed at our two sites. It seems that the remaining smaller shoots are not representative of the total shoot biomass; therefore, when they are included with the largest shoots for biomass prediction, they may significantly distort the resulting biomass estimates. In addition, since the 3 largest shoots are significantly bigger (the biggest one is clearly visible) than the remaining shoots, they can be easily identified within a plant and measured. This can also significantly accelerate biomass inventory of plantations and biomass estimation.
A number of methods for indirect woody biomass estimation in coppices have been published ([13], [40]), as direct methods of measurement are technically demanding and time consuming. However, the majority of indirect methods, like the assessment of biomass by the integration of remote sensing, have been developed for one- or few-stemmed trees and have to be adapted to the very fast-growing trees and short rotation periods of harvest ([4]). The presented approach, based on allometric equations using the averages of easily measurable parameters of 1-3 largest shoots, is less time-consuming with a sufficiently high accuracy; the DBH attribute seems to be the most suitable for the prediction of the amount of biomass. The growth models developed for assessing biomass volume, based on simple, non-destructive measurement of the stem diameter, may be useful in predicting biomass yields for assessing the economic viability of plantations and allow the study of allometric relationships of clonally replicated woody plants ([31], [5]). However, it is convenient to create allometric models based on sample trees of a specific clone, site and age ([9]). The age ([6], [24]), site ([11], [18]) and species ([42], [27]) factors can affect the allometric relationships used to predict the biomass yield. This finding was also confirmed in closely related species of the Salix genus ([19]).
We observed that the predicted total (summed) sample tree biomass was most accurate when using the DBH of the three largest shoots, with a deviation from the total weighed biomass of ≤ 0.06% only. This result corresponds with the finding of Šrámek et al. ([38]), who reported a sufficient accuracy with an error of ≤ 0.2% using the DBH of the three largest shoots in a later post-resprouting period of a traditional oak coppice. Likewise, Mosseler et al. ([19]) reported a more accurate estimation of shoot biomass using 3 shoots, compared with 20 in shrubs of the genus Salix. On the other hand, Matula et al. ([17]) reported that, in order to gain an accurate estimation of biomass (≤ 0.06%), it is necessary to use the basal diameter of two-to-five of the largest shoots. It seems that differences may exist in the relationship between the number of shoots used and the accuracy of estimates, depending on whether they are fast-growing trees, such as poplars and willows. For example, high estimation accuracy is obtained using the largest shoot at the stump level, while lower accuracy is obtained for biomass estimation at the 1 ha level.
Estimates of biomass at the stand level were compared with the real amount of biomass, which had been harvested and chipped on the plantations and transported to the heating plant where it was weighed. The difference between the observed amount of biomass and its prediction was calculated as a percentage deviation from the actual amount of biomass. However, a part of biomass was lost during the harvesting operations and remained in the field. The comparison did not take into account these losses during the transport of and manipulation with the wood chips, which can amount up to 5% of the total volume of the harvested biomass ([35]). Usually, the moisture loss in wood chips, and also biomass losses due to fungi and microbiological activities in piles at the depot (where the wood chips are stored until they are taken to the customer), should also be taken into consideration in the calculation ([34], [48]). The amount of dry mass loss can be from 0.5% up to 10% per month ([7], [33]), depending on the time when the wood chips are left at the depot as well as on climatic conditions and storage options. The deviation of the biomass estimate can therefore vary. However, in this study wood moisture changes during the transport and storage were not considered, as no statistically significant change in moisture was found until all wood chips were removed from both sites. Moreover, the chips were transported from the depot within 14 days. The deviation of the biomass estimate from the real amount was below 7% at Sobechleby and below 15% at Vícenice.
The reason for the relatively higher underestimation of biomass at the Vícenice plantation is not clear. It could have been caused by the stratified random selection of sampling plots within the plantation during the inventory. In specific cases, this may lead to the underrepresention of microsites where the amount of biomass on the stump may be significantly different (greater or smaller) from the rest of the plantation. The random sampling probably failed to fully capture more biomass produced on the stumps in microsites with greater nutrient or water availability. Therefore, the application of a regular network of inventory plots instead of stratified random sampling may be a more appropriate approach for biomass sampling in SRCs. This could minimise the omission of some types of microsite in large heterogeneous plantations.
Conclusions
Our results confirmed that the methodology applied for the estimation of biomass yield using allometric equations with averaged attributes of a few largest shoots, can be conveniently applied to poplar hybrid clone J-105. For a sufficient accuracy of biomass yield estimates in multi-stemmed trees of poplar SRC, we suggest to measure the DBH of the 3 largest shoots of each stump. Moreover, we illustrated how easily and quickly biomass yield can be estimated by measuring the DBH, and demonstrated that multi-stemmed trees can be simply included into ecosystem studies of woody vegetation with less time-consuming measurements.
Acknowledgements
We thank Verbava a.s. for their cooperation in selecting poplar SRC plantations and for providing information. We thank Michaela Kruttová for help with data collection. This work was supported by the Specific University Research Fund of the FFWT Mendel University in Brno (LDF_VP_2019031).
The authors declare no conflict of interests.
References
Gscholar
CrossRef | Gscholar
Gscholar
CrossRef | Gscholar
Gscholar
Gscholar
Gscholar
Gscholar
Gscholar
Gscholar
CrossRef | Gscholar
CrossRef | Gscholar
Gscholar
CrossRef | Gscholar
CrossRef | Gscholar
Gscholar
Authors’ Info
Authors’ Affiliation
Marie Matoušková 0000-0003-4780-4674
Klára Lengálová 0000-0001-8255-9729
Department of Forest Botany, Dendrology and Geobiocoenology, Mendel University in Brno, Zemedelská 3, 61300 Brno (Czech Republic)
Jaroslav Bubeník
Department of Phytoenergy, Silva Tarouca Research Institute for Landscape and Ornamental Gardening, Kvetnové nám. 391, Pruhonice, 252 43 (Czech Republic)
Department of Forest Ecology, Faculty of Forestry and Wood Sciences, Czech University of Life Sciences Prague, Kamýcká 129, 165 00 Prague (Czech Republic)
Corresponding author
Paper Info
Citation
Šrámek M, Weger J, Bubeník J, Matoušková M, Lengálová K, Matula R (2023). Effective woody biomass estimation in poplar short-rotation coppices - Populus nigra × P. maximowiczii. iForest 16: 202-209. - doi: 10.3832/ifor4200-016
Academic Editor
Petar Antov
Paper history
Received: Aug 10, 2022
Accepted: May 11, 2023
First online: Jul 25, 2023
Publication Date: Aug 31, 2023
Publication Time: 2.50 months
Copyright Information
© SISEF - The Italian Society of Silviculture and Forest Ecology 2023
Open Access
This article is distributed under the terms of the Creative Commons Attribution-Non Commercial 4.0 International (https://creativecommons.org/licenses/by-nc/4.0/), which permits unrestricted use, distribution, and reproduction in any medium, provided you give appropriate credit to the original author(s) and the source, provide a link to the Creative Commons license, and indicate if changes were made.
Web Metrics
Breakdown by View Type
Article Usage
Total Article Views: 353
(from publication date up to now)
Breakdown by View Type
HTML Page Views: 0
Abstract Page Views: 0
PDF Downloads: 317
Citation/Reference Downloads: 0
XML Downloads: 36
Web Metrics
Days since publication: 368
Overall contacts: 353
Avg. contacts per week: 6.71
Article Citations
Article citations are based on data periodically collected from the Clarivate Web of Science web site
(last update: Nov 2020)
(No citations were found up to date. Please come back later)
Publication Metrics
by Dimensions ©
Articles citing this article
List of the papers citing this article based on CrossRef Cited-by.
Related Contents
iForest Similar Articles
Research Articles
Allometric equations to estimate above-ground biomass of small-diameter mixed tree species in secondary tropical forests
vol. 13, pp. 165-174 (online: 02 May 2020)
Research Articles
Climate-wise models of biomass productivity for hybrid poplar clones in Europe
vol. 16, pp. 188-194 (online: 30 June 2023)
Research Articles
Energy production of poplar clones and their energy use efficiency
vol. 7, pp. 150-155 (online: 23 January 2014)
Research Articles
Allometric equations to assess biomass, carbon and nitrogen content of black pine and red pine trees in southern Korea
vol. 10, pp. 483-490 (online: 12 April 2017)
Research Articles
Field performance of poplar for bioenergy in southern Europe after two coppicing rotations: effects of clone and planting density
vol. 5, pp. 224-229 (online: 02 October 2012)
Research Articles
Allometric relationships for volume and biomass for stone pine (Pinus pinea L.) in Italian coastal stands
vol. 6, pp. 331-335 (online: 29 August 2013)
Research Articles
Verification of new Populus nigra L. clone improvement based on their performance over three rotations
vol. 13, pp. 185-193 (online: 12 May 2020)
Research Articles
Effects of planting density on the distribution of biomass in a douglas-fir plantation in southern Italy
vol. 8, pp. 368-376 (online: 09 September 2014)
Technical Reports
GlobAllomeTree: international platform for tree allometric equations to support volume, biomass and carbon assessment
vol. 6, pp. 326-330 (online: 18 July 2013)
Research Articles
Biomass production of Populus nigra L. clones grown in short rotation coppice systems in three different environments over four rotations
vol. 7, pp. 233-239 (online: 10 March 2014)
iForest Database Search
Search By Author
Search By Keyword
Google Scholar Search
Citing Articles
Search By Author
Search By Keywords
PubMed Search
Search By Author
Search By Keyword