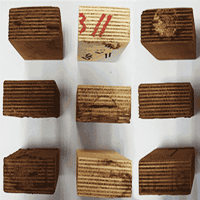
Heat treatment of poplar plywood: modifications in physical, mechanical and durability properties
iForest - Biogeosciences and Forestry, Volume 16, Issue 1, Pages 1-9 (2023)
doi: https://doi.org/10.3832/ifor4159-015
Published: Jan 09, 2023 - Copyright © 2023 SISEF
Research Articles
Abstract
Plywood made of poplar are limited to indoor usages since poplar exhibits a rather low natural durability. Recently, wood heat treatments have been applied to improve properties such as decay susceptibility and dimensional stability. This study examines the potential of exposing poplar plywood to heat treatment to extend the potential of applications of this engineered wood product to outdoor end uses, and new markets accordingly. Plywood panels were glued with two different adhesive formulations based on the same melamine-urea-formaldehyde (MUF) resin to compare their respective ability to resist to the heat treatment. These different plywoods were thermally modified in saturated steam conditions at 215 °C for 2 hours following the ThermoWood® process, up to reach 14% in mass loss. The durability improvement brought by the heat treatment was assessed in order to evaluate any possible outdoor uses for such plywood. After all the conducted analyses, the potential to use heat treated poplar plywoods in humid interior and protected exterior service conditions was confirmed.
Keywords
Heat Treatment, Plywood, Poplar, Bending Modulus of Elasticity, Bending Strength, Bond Quality, Fungal Durability, Termite Resistance
Introduction
As claimed in the 25th International Poplar Commission ([33]), poplar wood is widely produced in Europe and Northern America as raw material for a wide range of applications such as food packing crates, pulp, paper, engineering wood productions (EWP), furniture and even valuable non-wood products such as medicinal extracts and food products. Secondly, poplar as part of the fast-growing trees is of major interest as a renewable resource for the future green economy. Indeed, poplars are effective at sequestering carbon, thus contributing to the adaptation and mitigation of the climate change effects. Nonetheless, poplar wood shows a very low natural durability either against insects (Durability Class S-Sensible) or fungi (Durability Class 5-Not durable), according to the EN 350 standard ([7]), limiting the market range of this timber ([22], [14]) and any of its wood-products to indoor applications, corresponding to use class - 1 as specified in the EN 335 standard ([5]).
On the other hand, as summed up by Sandberg et al. ([46]), the market for innovative durable products made of modified wood has increased substantially during the last few years, especially in Europe. As a result, wood modification has been implemented to improve some intrinsic properties of wood, widen the range of sawn timber applications, and acquire the form and functionality desired by engineers, without bringing environmental friendliness into question. Several thermal modification technologies appeared recently, reflecting the increasing need to enhance the use of the ligno-cellulosic materials (reviewed by [17]). For instance, it can be mentioned the processes implying treatment kiln in open air ([25], [38]), with nitrogen as the “Retiwood®” or “Bois Retifié®” ([13], [14]), vacuum with the VacWood® process ([45]), steaming as in ThermoWood® or Perdure® ([28], [29], [39]), superheated steam as in PlatoWood® ([12], [8]) or processes using oil impregnation as in OHT® from Germany ([43], [44]) or the “Bois Perdure” treatment ([37]) in France. One of the most common commercial thermal modification processes, named ThermoWood®, started in 1993 in Finland by the International ThermoWood Association ([20]). All those heat-treatments allow to up-grade the natural durability of massive poplar wood from a durability class 5 “not durable” according to the EN 350 ([7]) and Gérard et al. ([23]), to a durability class 1-2 (very durable - durable - [15]), thus opening new markets for this thermally modified timber. Besides, as a fact, the mass loss after any heat treatment is widely considered as a good indicator of mechanical performances ([42]), durability towards insects ([14], [24]) and fungi ([30]).
Despite the great scientific interest focused on the modification occurring in massive woods thermally or chemically modified, a very low amount of work consider the heat treatment of EWP as a valuable economic solution. Sernek et al. ([47]) studied the bonding strength of 3 different adhesives (melamine-urea-formaldehyde (MUF), phenol-resorcinol-formaldehyde (PRF), and polyurethane (PUR) on 5 different European wood species after heat treatments using the PlatoWood® process showing the great ability of those glues to keep their gluing performances; later Andromachi & Ekaterini ([9]) studied the adhesive bond performance of heat treated fir wood but not as a heat treated EWP but only as a glue between modified woods. Nazerian & Dahmardeh Ghalehno ([40]) explored the mechanical and physical performances of laminated veneer lumbers (LVL) made of heat-treated poplar veneers in small heating unit at atmospheric pressure before being assembled into LVL as Sandak et al. ([45]) did on poplar veneers under vacuum convective kiln. In the same way, numerous researches ([52], [19], [10], [32], [35]) considered to heat treat veneers rather than the whole EWP.
Few authors looked at the thermal effect on EWP as ([48]) for fire resistance purposes rather than the use of this kind of product for exterior applications. Grinins et al. ([26]) considered the two possible approaches for producing thermally treated plywood: the first one being to treat the veneers separately before assembling them into the EWP; the second one being the treatment of the whole EWP. Afterwards, Lunguleasa et al. ([36]) considered, in an extensive experimental work, the possibility to thermally treat already glued beech plywood and to check the physical and mechanical properties of such a new product. A complementary research work by Sernek et al. ([47]) explored the adhesive bond shear strength modification after Plato® heat treatment of laminated beams made of different wood species (Norway spruce, Douglas fir, poplar, birch, and alder) glued with three different adhesives: MUF, PRF, and PUR. They demonstrated that MUF and PUR confer better bonding performances than PRF adhesive for thermally treated wood.
In the same trend than the above mentioned studies, the challenge of the present paper is to check the ability of a EWP such as plywood made of poplar to be heat treated in order to be used for outdoor applications. The ThermoWood® thermal modification process is the one performed in this work in collaboration with Bois Durable de Bourgogne (Vendenesse-lès-Charolles, France). For this purpose, several properties have been assessed among which the mechanical performances, the bonding strength of the glue after heat treatment, and the durability of the final product to conclude about its potential to be used in exterior.
Material and methods
Materials
Commercial plywood panels made of I-214 poplar and provided by Brugère factory (Chtillon-sur-Seine, France) were used in this study. The panels were composed of 21 layers up to a total thickness of 25 mm; each veneer being 1.2 mm thick. Two different MUF adhesive formulations from BASF© Kauramin type were used to build up the panels: a conventional formulation noted MUF69 based on its resin percentage content, and a diluted one noted MUF41 for the same reason. The composition of the two formula are described in Tab. 1. The spreading rate was the same for both formulation and equal to 200 g m-2.
Tab. 1 - Adhesive formulations: the composition is described in equivalent in mass (Mass eq., kg) and percentage of the total mass (Perc.).
Denomination | MUF69 | MUF41 | ||
---|---|---|---|---|
Mass eq. (kg) |
Perc. | Mass eq. (kg) |
Perc. | |
Resin BASF KAURAMIN 610 | 50 | 69.4 | 50 | 40.7 |
Water | 3 | 4.2 | 40 | 32.5 |
Wheat flour | 6 | 8.3 | 26 | 21.1 |
Olive pit powder | 6 | 8.3 | 0 | 0.0 |
Hardener BASF BONIT (10% dilution) | 7 | 9.7 | 7 | 5.7 |
A total of 64 specimens were sawn from at least 3 different plywood panels into a beam shape with 25 × 50 × 700 mm3 dimensions; half of the specimen were cut with the external veneers grain direction toward the length of the beams (“Parall.”) and the other half perpendicularly (“Perp.”). The specimens average Equilibrium Moisture Content (EMC) prior to heat treatment was 12.7% ± 0.3%, under stabilized 65% RH (Relative Humidity) and 20 °C; under such conditions, their average densities were 549 ± 6 and 502 ± 9 kg m-3, respectively for the MUF69 and the MUF41 panels. Then, 32 specimens (half Perp. and half Parall.) were exposed to the heat treatment (described in the next subsection) and 32 specimens (half Parall. and half Perp.) were kept as control and as reference of the non-treated plywood properties. The plywood panels glued with MUF69 have always a higher density than the ones by MUF41 by 9%.
Thermal modification
The ThermoWood® high-temperature treatment was carried out on the beam shape specimens at 215 ° C for 2 hours (for a total process duration of 29 hours) in a saturated water vapour atmosphere. Prior to the treatment, no dedicated conditioning were achieved out of the storage at the room conditions for at least 2 months. The treatment temperature-time curve is provided in Fig. 1a and the visual aspect of the treated specimen together with the control non-treated ones in Fig. 1b.
Fig. 1 - Heat treatment under saturated steam. (a) Description of the ThermoWood® heat treatment kinetic; (b) aspect and dimensions (in mm) of the beams before and after the treatment.
The heat treatment was performed up to an average (13.8% ± 0.5%) mass loss, as assessed from the mass measurements performed before and after the heat treatment on each specimen and described in the next subsection.
Physical properties
Samples dimensions after conditioning at 65% RH and 20 °C, using a climatic chamber (accuracy ± 0.5 °C and ± 1% RH), were measured by a calliper (± 10 µm) for the width and thickness, and a stainless-steel ruler (± 0.5 mm) for the length, before and after the thermal modification together with the mass which has been measured by an analytical balance with 0.01 g accuracy. The dimensional variations due to the treatment in the different directions was then calculated and the variation of density was also computed from mass and dimensions measurements. Since the heat treatment is lowering the wood equilibrium moisture content (EMC), the specimen moisture contents were measured before and after the heat treatment when they reached their hydric equilibrium under the same 65% RH and 20 °C conditioning.
Mechanical properties
The mechanical properties had been assessed after conditioning at 65% RH and 20 °C in climatic chamber. Each beam, heat treated and control ones, was tested in a three-points bending test until failure using a Zwick/Roell 250 kN static testing device. The bending test was set up with a distance equals to 16 times the specimen’s thickness between the supports in agreement with the standard EN 310 ([1]). The tests were performed with a cross-head speed so that the failure occurs within 60 ± 30 s. The bending strength -from the maximum load achieved Fmax - and the bending modulus of elasticity (MoE) - from a linear regression in the elastic part of the load/displacement curve between 0.1Fmax and 0.4Fmax - were extracted from each test using a Python routine.
Bonding performance
Bonding quality had been assessed according to standards EN 314-1 ([4]) and EN 314-2 ([2]) to investigate if the produced plywoods could fulfill the requirements of the bonding class 2. The test consists of a shear test on a bonding joint after pre-treatments. All bonding joints should normally be tested for certification, in this study only the bonding joints from either side of the middle central were tested. For bonding class 2, the two pre-treatments consist in: (i) Pre-treatment 5.1.1: immersion for 24 h in water at 20 °C; (ii) Pre-treatment 5.1.2: immersion for 6 h in boiling water followed by a cooling in water at 20 °C for 1 h.
For each pre-treatment, 10 samples have been tested in shear. The load was applied at a constant rate of motion so that rupture occurs within 20 and 40 s. The shear strength (fv) is calculated from the following eqn. 1:
where F is the maximum load in Newton, l and b the dimensions in millimetres of the shear area (measured prior to the different pre-treatment). For the 10 tested specimens, and for each pre-treatment, the average shear strength was calculated. To be conclusive for a bonding class 2 quality, the average shear strength needs to be higher than 1 MPa.
Durability assessment
Fungal resistance
Decay resistance tests were adapted from the guidelines from the standard XP ENV 12038 ([3]), with some adjustments concerning the samples sizes and the choice of wood destroying fungi. For each plywood modality, 2 batches composed by 18 samples from 3 different panels (50 × 48 × 25 mm) were exposed to Trametes versicolor (TV - Linnaeus, CTB 863 A) and Rhodonia placenta Coocke sensu J. Erikson (RP - Fries, FPRL 280), grown on malt/agar medium (malt 40 g L-1, agar 20 g L-1). Ten solid wood blocks (50 × 25 × 15 mm, L × R × T) of both beech (Fagus sylvatica) and pine (Pinus sylvestris) sapwood were tested as virulence control (Control-Vir). Six blocks of both beech and pine sapwood with the same dimensions as the plywood specimens were also exposed to the fungi as size control samples (Control-Dim). All specimens were sterilized by γ-radiations prior to fungal exposure. After 16 weeks at 22 ± 1 °C, 75 ± 5% RH of the fungal exposure, the block specimens were dried at 103 °C and their final weight (m2) was recorded. Finally, weight losses (WL, %) were determined as a percentage of the initial anhydrous weight (m1) as in eqn. 2:
If the average mass losses are greater than 3% (m/m), the Degradation Sensitivity Index (DSI) was determined as in eqn. 3:
where T is the weight loss of a test specimen (in %), and S the average weight loss of the series of size control specimens (in %).
Termite resistance
Termite resistance tests were adapted from the guidelines of standard EN 117 ([6]), with some adjustments concerning the sample sizes. Untreated and heat-treated plywood samples of dimensions (50 × 48 × 25 mm) were exposed to Eastern subterranean termites (Reticulitermes flavipes, ex. santonensis de Feytaud) in non-choice and choice tests (Fig. 2).
Fig. 2 - Termite resistance tests of untreated and heat-treated poplar plywood samples: (a) non-choice test devices and (b) choice test devices.
Termites were collected from Oleron Island, France (45° 49′ 05.9″ N, 01° 13′ 47.8″ W). The colony was reared in a climatic chamber regulated at 27 °C and RH >75%. Five beech (Fagus sylvatica) and five pine sapwood (Pinus sylvestris) samples with dimensions of 50 × 25 × 15 mm (L × R × T) were also tested against termite as virulence controls.
Before exposure to termites, plywood and control samples were dried at 103 °C in order to determine their anhydrous initial weight (m3), and then re-conditioned at 20 °C, 65% RH until constant weight. For each plywood modality, 3 replicates were tested for non-choice (one plywood samples per test device) as well as choice tests (one untreated and one heat treated plywood samples with the same glue per test device), respectively. A total of 250 termite workers, 3 nymphs and 3 soldiers were then introduced into each test device, i.e., 9 cm diameter glass flask containing a 5 cm thick layer of wet sand (4 sand / 1 water, v/v). These test devices were placed for 8 weeks in a dark climatic chamber conditioned at 27 °C and RH >75%, and were regularly watered and checked. At the end of the exposure, the samples were cleaned of sand and the termite alive were counted. Visual ratings were attributed to samples, according to the criteria of EN 117 ([6]), with adjustments to the sample size. Then, the samples were dried at 103 °C to obtain their final anhydrous weight (m4), and their mass losses (ML, %) were calculated as in eqn. 4:
Results and discussion
Physical properties modification after heat treatment
The dimensions and density evolution of the sample due to the heat treatment were assessed and are summarized in Tab. 2, where the relative difference between the average values of the treated specimen compared to the control (non-treated) ones are reported. Measures were obtained after specimen conditioning at 65% RH and 20 °C and oven drying (103 °C) when necessary, i.e., EMC determination, and ML at 0% of moisture content (noted MC).
Tab. 2 - Evolution of the dimensions, density, and equilibrium moisture content (EMC) of the specimen after the heat treatment. Values are average ± standard deviation of the relative differences (Δ) between the values of the treated specimen compared to the control non-treated ones. (*): see Tab. 3for details by sampling groups; (**): for all samples (MUF69 and MUF41 together). “Standard” stands here for conditioning at 65% RH and 20 °C.
Parameter | Conditions | MUF69 | MUF41 | ALL** |
---|---|---|---|---|
Δ thickness (%) | Standard | -1.96 ± 0.32 | -1.23 ± 0.32 | -1.54 ± 0.57 |
Δ width (%) | Standard | -0.01 ± 0.14 | -0.09 ± 0.25 | -0.09 ± 0.19 |
Δ length (%) | Standard | -0.14 ± 0.05 | -0.09 ± 0.03 | -0.10 ± 0.06 |
Δ mass (%) | Standard | -13.90 ± 0.30 | -13.80 ± 0.70 | -13.80 ± 0.50 |
Δ mass (%) | Oven dry | -11.40 ± 0.40 | -10.90 ± 0.70 | -11.20 ± 0.60 |
Δ density * (%) | Standard | -12.40 ± 0.20 | -13.10 ± 0.70 | -12.60 ± 0.70 |
Δ EMC* (%) | Standard | -24.90 ± 0.30 | -28.20 ± 0.20 | -26.80 ± 0.30 |
The dimensions measurements showed that for plywood, being composed of an arrangement of veneers glued perpendicularly to each other, the in-plane variations (standing for tangential and longitudinal anatomical directions of the wood) are prevented since this variation is below 0.2%. Only the thickness (radial direction of the veneers) of the specimen is quite affected by thermal modification by almost 1.5% when compared to the initial thickness of the specimen. As for comparison, Marcon et al. ([38]) provided dimensional shrinkages for massive poplar wood (Populus alba L.) heat treated in conventional oven under different temperatures and durations. For an equivalent 11.2% oven dry mass loss (average of MUF69 and MUF41 samples mass losses chosen as representative of the heat treatment global intensity), the percentages of shrinkage were about 1.4%, 2.8%, and below 0.05%, respectively for the radial, tangential, and longitudinal anatomical directions (data from the kinetic model of [38], not the experimental data). Thus, the radial shrinkage (thickness of the specimen) observed in the present study for an oven dry mass loss of 11.2% agrees with the results. In the same way, as the shrinkage due to thermal modification is rather low in the longitudinal anatomical direction, the in-plane shrinkage observed here are equivalent to the longitudinal one reported by Marcon et al. ([38]), though slightly higher. It is worth noting that the fibres act as shrinkage preventer on both direction thanks to the 90° plywood assembly.
Additionally, Marcon et al. ([38]) provided a density reduction of about 8.8% for 11.2% of mass loss, which is slightly lower than the density reduction observed in the present study (about 12.6%). This could be due to the presence of the glue, which seems to be more affected in term of density than the wood, even though MUF resins are thermosetting glues. Indeed, Liu et al. ([34]) showed that MUF adhesives after polimerization can still experience extended thermal modifications above 200 °C, which result for instance by the isocyanates cycles degradation (part of the mass loss observed) eventually responsible for the reduction of the bonding quality.
Concerning the equilibrium moisture content (EMC), as summarized in Tab. 3 under conditioning at 65% RH and 20 °C, the EMC reduction by 28.2% of the treated sample compared to the non-treated (controls) for the MUF41 formulation is slightly higher than for the MUF69 formulation (24.9%).
Tab. 3 - Summary of the four sampling sets with their respective descriptive properties before the thermal modification to assess their initial statistical homogeneity. Density and equilibrium moisture content are provided after conditioning at 65% RH and 20 °C. (N): number of assayed specimens.
Orientation | Glue | Heat treatment | N | Before heat treatment | |
---|---|---|---|---|---|
Density (kg m-3) |
EMC (%) |
||||
Parall. | MUF69 | No | 8 | 541 ± 3 | 12.8 ± 0.1 |
Yes | 8 | 540 ± 6 | 9.6 ± 0.2 | ||
MUF41 | No | 8 | 502 ± 14 | 12.7 ± 0.1 | |
Yes | 8 | 502 ± 12 | 9.0 ± 0.1 | ||
Perp. | MUF69 | No | 8 | 557 ± 7 | 12.7 ± 0.6 |
Yes | 8 | 556 ± 6 | 9.5 ± 0.1 | ||
MUF41 | No | 8 | 502 ± 7 | 12.6 ± 0.1 | |
Yes | 8 | 502 ± 5 | 9.1 ± 0.3 | ||
Totals | - | Total untreated | 32 | 526 ± 26 | 12.7 ± 0.3 |
Total treated | 32 | 525 ± 25 | 9.3 ± 0.3 |
Similarly, the massive poplar wood study reported by Marcon et al. ([38]) showed that the EMC at 65% RH and 20 °C was reduced by 39.6% (from 8.5% for the non-treated massive wood to 5.2% for the treated one) for an oven dry mass loss of 11.2%. In comparison, the poplar plywood modification applied in the present study reduces the EMC by 26.8% in average (both glues) for the same oven dry mass loss of 11.2%. As previously metioned, the above discrepancy could result from the presence of the glue, whose hygroscopicity is less affected than the massive wood, thus resulting in a higher EMC for thermo-treated plywood at standard conditions (65% RH and 20 °C) compared to a thermo-treated solid wood at such conditioning.
Mechanical properties variations
The results in Tab. 4 show how heat treatments can quickly affect the mechanical performances of wood products. The strength is the main mechanical property which was reduced (on average by 56% and 48% for the “Parall.” and the “Perp.” specimens, respectively). On the contrary, the bending MoE had been reduced by only 5% on average, with no differences due to the glue nor the direction the specimen had been cut from the full plywood panel.
Tab. 4 - Modifications of mechanical properties at 65% RH and 20 °C. Values are provided as mean ± standard deviation (SD) and the relative difference (Δ, %) between the average values of the treated specimen compared to the control non-treated ones. (HT): Heat treatment; (*): for all samples (Parall. and Perp. together); (**): data measured at oven dry state (0% MC), and for an oven dry ML of 11.2% being the same than in the measured data of the present study; (***): correction of the stiffness values from 0% MC to respectively 12.0% MC for the non-treated values and 9.3% MC for the treated ones thanks to formulas provided in ([27]). The methodology is described in details in Viguier et al. ([50]).
Bending MoE |
Orientation | Glue / Type | HT | Bending MoE | Bending strength | ||
---|---|---|---|---|---|---|---|
MPa (mean ± SD) |
Δ (%) |
MPa (mean ± SD) |
Δ (%) |
||||
3-points bending (65% RH and 20 °C) |
Parall. | MUF69 | No | 5156 ± 209 | -2.3 | 48.5 ± 2.8 | -54 |
Yes | 5035 ± 282 | - | 22.5 ± 3.1 | - | |||
MUF41 | No | 4619 ± 268 | -5.9 | 40.1 ± 2.6 | -58 | ||
Yes | 4909 ± 288 | - | 16.8 ± 2.2 | - | |||
Perp. | MUF69 | No | 4618 ± 169 | -7.8 | 41.5 ± 2.7 | -43 | |
Yes | 4257 ± 204 | - | 23.7 ± 2.4 | - | |||
MUF41 | No | 3976 ± 324 | -5.2 | 37.4 ± 4.0 | -53 | ||
Yes | 3768 ± 372 | - | 17.6 ± 2.7 | - | |||
ALL* | MUF69 | No | 4887 ± 329 | -9 | 45.0 ± 4.4 | -14 | |
MUF41 | No | 4443 ± 558 | - | 38.7 ± 3.7 | - | ||
MUF69 | Yes | 4646 ± 460 | -10 | 23.1 ± 2.9 | -26 | ||
MUF41 | Yes | 4194 ± 535 | - | 17.2 ± 2.5 | - | ||
3-points bending (0% MC, oven dry) |
Longitudinal | Poplar Solid Wood ([38])** |
No | 8570 | -16 | - | - |
Yes | 7180 | - | - | - | |||
Radial | No | 660 | -24 | - | - | ||
Yes | 504 | - | - | - | |||
Tangential ER/ET = 2.05 |
No | 388 | -24 | - | - | ||
Yes | 296 | - | - | - | |||
3-points bending (65% RH and 20 °C) |
Longitudinal | Poplar Solid Wood Corrected*** |
No | 6937 | -11 | - | - |
Yes | 6178 | - | - | - | |||
Tangential | No | 373 | -23 | - | - | ||
Yes | 288 | - | - | - | |||
Parall. | Recomposed plywood*** (21 plies) |
No | 4124 | -11 | - | - | |
Yes | 3653 | - | - | - | |||
Perp. | No | 3187 | -12 | - | - | ||
Yes | 2813 | - | - | - |
Moreover, the MUF69 (whatever the direction considered) showed greater performances than the MUF41, as both the bending MoE and the strength were always better for MUF69: on average, by about 9% for the bending MoE and about 14% for the strength before the heat treatment; they become in relative average 10% and 25% respectively after the thermal modification keeping the same trend. The thermal treatment affected to a greater extend the MUF41 formulation than the MUF69 when focusing on strength; the difference between MUF41 and MUF69 bending MoE being almost unchanged both before and after the thermal treatment. If one considers that the two glue formulations are not affected in the same way, then it makes sense that the shearing along with the beams are not exhibiting the same distribution. The assumption that the MUF41 formulation is more weakened by the thermal treatment is supported by the bonding performance assessments (Tab. 5) showing its shear strength almost reduced to zero (-95%), while the MUF69 kept 43% of its original shear strength capacity (prior the heat treatment).
Tab. 5 - Bonding performance modification in terms of shear strength after two different pre-treatment. Values are provided absolute values (mean ± standard deviation, SD) and the relative difference (Δ, %) between the average values of the treated specimens compared to the control non-treated ones.
Pretreatment | Formulation | Heat treatment | Shear strength | |
---|---|---|---|---|
MPa (mean ± SD) | Δ (%) | |||
5.1.1 | MUF69 | No | 2.43 ± 0.33 | -57 |
Yes | 1.04 ± 0.32 | |||
MUF41 | No | 1.73 ± 0.38 | -95 | |
Yes | 0.09 ± 0.15 | |||
5.1.2 | MUF69 | No | 1.96 ± 0.35 | -53 |
Yes | 0.93 ± 0.17 |
The results reported by Marcon et al. ([38]) at oven dry state can help to understand whether the worsen bending mechanical properties are due to the wood itself or to some adhesive degradation during the heat treatment. Indeed, Marcon et al. ([38]) found, for the identical oven dry mass loss of 11.2%, a 3-points bending MoE reduction of about 16% in longitudinal direction and of about 24% in the radial one. Regarding the strength, the reduction in mechanical performances were of 50% and 44% respectively in longitudinal and radial anatomical directions.
Nonetheless, those data from the literature are given at oven dry state; a correction has to be applied to compare those data with the 65% RH and 20 °C conditions, which was done using the formulas provided in Guitard ([27]) at 12.7% MC and 9.3% MC for untreated and treated samples, respectively. Assuming that the anisotropy ratio ER/ET is equal to 1.7 in both poplar wood ([22]) and standard hardwoods ([27], referred as feuillu standard in the book), the tangential stiffness can be assessed. Afterward, two virtual poplar plywoods, one Parall. and one Perp., and their respective equivalent bending MoE can be computed following the Viguier et al. ([50]) method with the relative stiffness values of each ply considering its own material orientation and distance to the 3-points bending neutral axis (here at half the thickness). To conclude, as summarized on Tab. 4, the virtual plywood (without glue effect) is affected in the same manner than the actual plywood by the thermal treatment (at least up to an oven dry ML of 11.2%), since their bending MoE are lessened respectively by -11.6% and -9.5%; no significant influence on the adhesives is evidenced here.
Bonding performance
The results in Tab. 5 show how heat treatments affect drastically the bonding performance of plywood. Indeed, every non-heat-treated specimen reach the requirements of the standards for a bonding class 2, the average shear strength being greater than 1 MPa in every case. In the case of heat-treated plywood, specimen glued with MUF69 are approaching but not fulfilling the requirements for the bonding class 2, the average shear strength being higher than 1 MPa for 5.1.1 pre-treatment and quite close to 1 in the case of the 5.1.2 pre-treatment. The loss of shear strength was however rather high between the heat-treated and untreated samples (more than 100% loss). Concerning the plywood glued with MUF41, it was far from reaching the requirements; some of the ten specimens were even completely delaminated after the pre-treatment, therefore, test with pre-treatment 5.1.2 was not done.
The above results highlight the importance of the adhesive formulation, as heat treatment drastically affects the bonding formulation. Nevertheless, some industrial formulation seems to be already suitable for use in a bonding class 2 but without warranty of their residual performances after heat treatment.
Plywood resistance towards fungi
The effect of ThermoWood® treatment and the glue used for poplar plywood processing on decay resistance are reported in Fig. 3 and Fig. 4 (see also Fig. S1 in Supplementary material).
Fig. 3 - Fungal colonization of the Pine Control-Dim, Beech Control-Dim, MUF69 untreated and heat-treated poplar panels, and MUF41 untreated and heat-treated poplar panel samples, after 16 weeks of Rhodonia placenta exposition.
Fig. 4 - Weight loss occurred after decay resistance tests of control, untreated and heat-treated poplar plywood samples, against (a) Rhodonia placenta and (b) Trametes versicolor fungi. Humidity of control and plywood samples after 16 weeks of (c) Rhodonia placenta and (d) Trametes versicolor exposure.
The average weight losses for the virulence controls samples were 47.69% (Pine Control-Vir) and 36.55% (Beech Control-Vir) for Rhodonia placenta, while it was 22.26% (Pine Control-Vir) and 28.86% (Beech Control-Vir) for Trametes versicolor. These weight losses higher than 20% for each modality confirmed the good virulence of both strains and validated the decay resistance tests. The weight losses of the dimension controls (33.55% and 23.56% in Pine Control-Dim and Beech Control-Dim, respectively, with Rhodonia placenta, and 22.67% in Beech Control-Dim for Trametes versicolor) show that the plywood configuration does not affect the susceptibility to fungal attack, all weight losses being above 20%.
The Beech Control-Dim and Pine Control-Dim samples were totally recovered by both mycelia during the fungi tests; in comparison, the untreated and the thermally modified poplar panel samples were only partially colonized by the mycelium (Fig. 3).
Fig. 4 and Fig. S1 (Supplementary material) confirm the significant impact of ThermoWood® treatment on the decay resistance of poplar plywood, based on the weight loss (WL < 2%) and the visual aspect of the decayed samples. For both fungi, the average values of WL of non-treated MUF41 formulation and their thermally modified ones (MUF41-TT) are higher than the other formulation (MUF69 and MUF69-TT). This difference can be attributed to the glue concentration in MUF69 (as MUF41 is diluted MUF), a higher concentration in melamine being correlated to an increased fungal resistance ([49]).
In addition, thermal modification process reduced this difference, as MUF41-TT and MUF69-TT plywood samples had a similar fungal resistance, though a slightly higher WL average value was observed for MUF41-TT (0.99% for RP, 1.38% for TV) than those of MUF69-TT (0.71% for RP, 0.73% for TV). Yet, MUF69-TT samples had lower weight loss than those of MUF41-TT, thus both heat-treated poplar plywoods could be considered as durable (WL < 3%).
Fig. 4 shows that TV caused higher degradations on MUF69 and MUF41 poplar panel samples than RP, even if an opposed tendency is observed concerning the final humidity of the corresponding samples (Fig. 4c, Fig. 4d). The same consideration can be done concerning MUF69-TT and MUF41-TT, but the difference was less significant. Other past studies highlighted that white rot (Trametes versicolor) are more aggressive against hardwood species than brown rot (Rhodonia placenta - [11]). Similar conclusions were also found on thermally modified poplar wood ([15]).
ThermoWood® process, performed at 215 °C for 2 hours, resulting in mass loss (due to the wood thermal degradation) of the plywood samples of 14%, allow improving considerably the decay resistance of MUF69-TT and MUF41-TT material, until reaching an average value of weight loss (WL) lower than 1.38% (Fig. 4). According to the fungi tested in this study and the EN 350 ([7]) specifications for plywood, MUF69-TT and MUF41-TT have a durability class-1 (WL< 5% for RP and TV), whereas MUF69 and MUF41 have a durability class-4 (15% < WL <30% for TV) and -5 (WL> 30% for TV), respectively.
Previous studies showed slightly lower performance of thermal modification performed at a same intensity level (similar mass loss around 14%) on the durability of poplar wood block ([13], [31]). The high durability performances conferred to the MUF69-TT and MUF41-TT plywood samples, tested in this study, are probably due to the combination of glue concentration (to a small extend) and thermal modification (to a greater extend).
The results about decay resistance are particularly interesting, because poplar wood is not naturally durable and thermal modification process makes it suitable for the exposure to some humid conditions ([21], [51]), offering new perspectives of valorization for poplar plywood materials.
Resistance against termites
The effects of the heat-treatment and glue used for the elaboration of poplar plywood on termite resistance are reported in Tab. 6. The pine and beech control samples were severely degraded (mass loss up to 6.12% and 3.88%, respectively) with a termite survival rate greater than 62.67%; and both control samples have a visual rating of 4. These results confirmed the high virulence of termites and the validity of the termite resistance tests. According to the non-choice and choice tests, it appears that thermally modified plywood samples are more degraded than their respective untreated samples. Past studies showed that thermally modified wood can be more attractive for termites even if a higher mortality rate at the end of the test is observed than those of untreated wood ([41], [16]).
Tab. 6 - Termite resistance of untreated and heat-treated poplar plywood samples using non-choice and choice tests. (HT): Heat treatment; (SD): standard deviation; (*): 0, no attack; 1, attack attempt; 2, slight attack; 3, average attack; 4, strong attack. The number of samples is in brackets.
Tests | Sample references | HT | Mass Loss | Survival Rate | Visual Rating * |
---|---|---|---|---|---|
(%, mean ± SD) | (%, mean ± SD) | ||||
Non-choice tests | MUF69 | No | 0.94 ± 0.19 | 3.07 ± 5.31 | 3 (3) |
Yes | 1.17 ± 0.09 | 0.00 ± 0.00 | 3 (3) | ||
MUF41 | No | 1.14 ± 0.78 | 0.67 ± 1.15 | 3 (2) - 4 (1) | |
Yes | 1.65 ± 0.61 | 2.40 ± 4.16 | 3 (2) - 4 (1) | ||
Pine Control | No | 6.12 ± 0.80 | 65.68 ± 3.89 | 4 (5) | |
Beech control | No | 3.88 ± 0.57 | 62.67 ± 3.11 | 4 (5) | |
Choice tests | MUF69 | No | 1.61 ± 0.68 | 45.47 ± 12.29 | 3 (1) - 4 (2) |
Yes | 1.71 ± 0.69 | 4 (3) | |||
MUF41 | No | 0.86 ± 0.37 | 11.60 ± 19.75 | 2 (1) - 3 (1) - 4(1) | |
Yes | 1.82 ± 0.79 | 4 (3) |
After exposition to termites in non-choice tests, MUF41 and MUF41-TT plywood samples presented a similar maximal visual rating of 4 (Sensible), with a termite survival rate of 0.67% and 2.14%, and weight losses of 1.14% and 1.65%, respectively. In the same test conditions, MUF69 and MUF69-TT plywood samples showed a maximal visual rating of 3 (Sensible), with a termite survival rate of 3.07% and 0.00%, and weight losses of 0.94% and 1.17%, respectively. For each modality, more than 50% of the replicates had a visual rating of 3-4, that means that all poplar plywood (both untreated and both treated) are classified as sensible towards termite according to EN 350 ([7]).
Concerning the choice tests, similar conclusions can be stated. For each modality, more than 50% of the replicates had a visual rating of 3-4, that means that all poplar plywood (both untreated and treated) are still classified as sensible towards termite exposure according to EN 350 ([7]).
In terms of mass loss, all the plywood samples were less degraded than the control samples. All plywood samples showed visual ratings 3 or 4, reflecting average and severe attacks, respectively. In other words, heat-treatment process and glue concentration have no a major impact on plywood termite resistance improvement. As observed in this study, there is no significant effect of heat-treatment on heat-treated plywood samples and it appeared that the termite resistance of heat-treated wood was impacted in a random way, was indiscriminate and even reduced in many cases, according to modification process conditions ([14]).
In addition, the presence of formaldehyde within the two-tested glue seems to have no influence on the termite resistance of the poplar plywood panel. On the first hand, the termites usually do not (or at a very little scale) attack the glue lines, but they degrade mainly the wood veneers ([18]). On the other hand, formaldehyde is a volatile compound (VOC) and a high amount is probably removed during thermal modification process ([18]). Both these explanations could explain the fact that glues based on formaldehyde have no impact on the termite resistance of untreated and heat-treated poplar plywood. Finally, as observed in Fig. S2 (Supplementary material), the samples are mainly degraded on the exterior plies or by cavity occurred in a wood ply.
Conclusions
The ThermoWood® process allows improving considerably the decay resistance of MUF69 and MUF41 poplar plywood. These results are particularly interesting, because poplar wood is not naturally durable and thermal modification process makes it suitable for use in humid conditions, offering new perspectives of valorisation for poplar plywood materials. However, thermal modification of poplar plywood is not effective against termites. With or without thermal modification, and whatever the formaldehyde concentration within the glue formulations, all plywoods present a high number of tested samples with visual rating of 3 and 4, that means that all plywoods are sensible towards termite attacks.
These durability outcomes have to be mitigated regarding the physical and mechanical degradations assessments performed, since they are affected by the process as a common consequence of thermal modification treatments. Indeed, the strength and bonding performances are both reduced by about 50% (particularly, by about 100% for the MUF41 glue bonding strength). On the other hand, the bending MoE performance is only reduced by about 6%.
To resume, such thermal modification process is viable to upgrade poplar plywood fungal resistance, and will allow to shift its service from indoor to outdoor, above-ground, in protected condition or short period of weathering (no water accumulation) as long as they are not used as structural elements.
List of abbreviations
EMC: equilibrium moisture content in %; MC: moisture content in %; RH: relative humidity in %; Parall. and Perp.: design the first and last plies grain orientation toward the 3-points bending solicitation; CP: stands for plywood; MUF: designs an adhesive formulation of a melamine-urea-formaldehyde glue; PRF: phenol-resorcinol-formaldehyde adhesive; PUR: polyurethane adhesive; EWP: engineering wood product; TT: thermo treated; WL: weight loss; ML: mass loss; TV: fungi type Trametes versicolor (Linnaeus, CTB 863 A); RP: fungi type Rhodonia placenta Coocke sensu J. Erikson (Fries, FPRL 280).
Acknowledgements
The authors acknowledge the company Bois Durables de Bourgogne, and particularly Jean Perche, for its contribution in the thermal modification processes. This study was performed thanks to the partnership of BOPLI (⇒ https://artsetmetiers.fr/fr/bopli-bourgogne-placage-innovation). The authors would also like to thank the Xylomat Technical Platform from the Xylomat Scientific Network, funded by ANR-10-EQPX-16 XYLOFOREST.
References
Gscholar
Gscholar
Gscholar
Gscholar
Gscholar
Gscholar
Gscholar
Online | Gscholar
CrossRef | Gscholar
CrossRef | Gscholar
Online | Gscholar
Gscholar
CrossRef | Gscholar
Gscholar
Gscholar
Gscholar
Gscholar
Gscholar
Gscholar
Gscholar
Gscholar
Authors’ Info
Authors’ Affiliation
Joffrey Viguier 0000-0001-7926-599X
Jean-Claude Butaud
Louis Denaud 0000-0002-4370-6076
Robert Collet 0000-0001-8213-8959
Arts et Metiers Institute of Technology, LaboMaP, UBFC, HESAM, Cluny (France)
Marie-France Thevenon 0000-0003-2985-2728
Luc Pignolet
Adélaïde Gartili
CIRAD, UPR BioWooEB, F-34398 Montpellier (France)
Marie-France Thevenon 0000-0003-2985-2728
Luc Pignolet
Adélaïde Gartili
BioWooEB, Univ Montpellier, CIRAD, Montpellier (France)
Corresponding author
Paper Info
Citation
Marcon B, Viguier J, Candelier K, Thevenon M-F, Butaud J-C, Pignolet L, Gartili A, Denaud L, Collet R (2023). Heat treatment of poplar plywood: modifications in physical, mechanical and durability properties. iForest 16: 1-9. - doi: 10.3832/ifor4159-015
Academic Editor
Manuela Romagnoli
Paper history
Received: Jun 16, 2022
Accepted: Nov 07, 2022
First online: Jan 09, 2023
Publication Date: Feb 28, 2023
Publication Time: 2.10 months
Copyright Information
© SISEF - The Italian Society of Silviculture and Forest Ecology 2023
Open Access
This article is distributed under the terms of the Creative Commons Attribution-Non Commercial 4.0 International (https://creativecommons.org/licenses/by-nc/4.0/), which permits unrestricted use, distribution, and reproduction in any medium, provided you give appropriate credit to the original author(s) and the source, provide a link to the Creative Commons license, and indicate if changes were made.
Web Metrics
Breakdown by View Type
Article Usage
Total Article Views: 868
(from publication date up to now)
Breakdown by View Type
HTML Page Views: 0
Abstract Page Views: 0
PDF Downloads: 672
Citation/Reference Downloads: 1
XML Downloads: 195
Web Metrics
Days since publication: 658
Overall contacts: 868
Avg. contacts per week: 9.23
Article Citations
Article citations are based on data periodically collected from the Clarivate Web of Science web site
(last update: Nov 2020)
(No citations were found up to date. Please come back later)
Publication Metrics
by Dimensions ©
Articles citing this article
List of the papers citing this article based on CrossRef Cited-by.
Related Contents
iForest Similar Articles
Short Communications
Influence of thermo-vacuum treatment on bending properties of poplar rotary-cut veneer
vol. 10, pp. 161-163 (online: 13 June 2016)
Research Articles
Physical-mechanical properties and bonding quality of heat treated poplar (I-214 clone) and ceiba plywood
vol. 8, pp. 687-692 (online: 17 December 2014)
Research Articles
Physical and mechanical characteristics of poor-quality wood after heat treatment
vol. 8, pp. 884-891 (online: 22 May 2015)
Research Articles
The physicomechanical and thermal properties of Algerian Aleppo pine (Pinus halepensis) wood as a component of sandwich panels
vol. 15, pp. 106-111 (online: 21 March 2022)
Research Articles
Hardness and contact angle of thermo-treated poplar plywood for bio-building
vol. 14, pp. 274-277 (online: 29 May 2021)
Research Articles
Testing common hornbeam (Carpinus betulus L.) acetylated with the Accoya method under industrial conditions
vol. 10, pp. 948-954 (online: 19 December 2017)
Research Articles
Improving dimensional stability of Populus cathayana wood by suberin monomers with heat treatment
vol. 14, pp. 313-319 (online: 01 July 2021)
Research Articles
Kinetic analysis of poplar wood properties by thermal modification in conventional oven
vol. 11, pp. 131-139 (online: 07 February 2018)
Research Articles
Physical, chemical and mechanical properties of Pinus sylvestris wood at five sites in Portugal
vol. 10, pp. 669-679 (online: 11 July 2017)
Review Papers
Moisture in modified wood and its relevance for fungal decay
vol. 11, pp. 418-422 (online: 05 June 2018)
iForest Database Search
Google Scholar Search
Citing Articles
Search By Author
Search By Keywords
PubMed Search
Search By Author
Search By Keyword