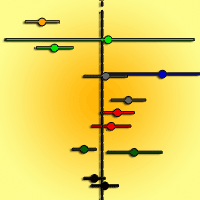
Could cattle ranching and soybean cultivation be sustainable? A systematic review and a meta-analysis for the Amazon
iForest - Biogeosciences and Forestry, Volume 14, Issue 3, Pages 285-298 (2021)
doi: https://doi.org/10.3832/ifor3779-014
Published: Jun 08, 2021 - Copyright © 2021 SISEF
Research Articles
Abstract
Tropical forests are being destroyed to make space for agricultural activities with the assumption that they are required to feed the growing global population. Consequently, more sustainable practices are needed to guarantee food security and environmental protection of highly threatened natural biodiversity hotspots like the Amazon rainforest. Cattle ranching and soybean cultivation are by far the greater drivers of land use change and deforestation in the Amazon region. We performed a systematic review of papers related to these two main drivers and a meta-analysis on the effects of sustainable practices on different ecosystem services. The results of the review highlight a large concern about the negative impacts of cattle ranching and soybean crops on the ecosystem dynamics and functionality of the Amazon biome, in addition to the clear relationship with deforestation. Another relevant finding is the large gap in empirical research concerning the effects of sustainable practices on different ecosystem services. Such a gap is evident since only 13 studies from the initial database met the requirements for a meta-analysis. Of the 171 comparisons between the ecosystem services provided in conventional land-uses and those adopting sustainable practices, the overall model indicated a non-significant effect, although the results were heterogeneous. Crop yield and herbage biomass were negatively affected, while livestock productivity, soil organic carbon, soil fertility and woody biomass were positively affected. Also, the six sustainable practices evaluated showed different outcomes, from a predominance of positive effects in silvopastoral systems, to a predominance of negative effects on agrosilvicultural systems. Our systematic review and meta-analysis indicate that cattle ranching and soybean cultivation can indeed be conducted in a more sustainable way, enhancing the provision of ecosystem services while avoiding deforestation. In turn, our results also highlight the lack of empirical data and the need to standardize the methodologies used to deeply assess the effects of such practices. In conclusion, we suggest a way to advance research into the real effects of sustainable practices aimed at reducing the negative impacts of cattle ranching and soybean crops in the Amazon.
Keywords
Tropical Forest, Agroforestry, Ecosystem Services, Silvopastoral Systems, Sustainable Practices, Cattle, Soybean
Introduction
Tropical forests harbor most of global terrestrial biodiversity and provide essential ecosystem services (ES), such as carbon sequestration ([112]), flood and drought control ([77]), influence on pluviometric regime ([37]) with resulting effects on climate at the global level ([71]). Currently, the major pressure on native global forests burdens the tropical region, mainly in the Amazon biome ([75]), where high rates of forest losses are often related to two main indirect factors: urban growth (rural-urban migration, urban food market) within countries ([22]) and growth of agricultural exports to other countries ([55], [95]). Changes in dietary preferences of the growing global population and the consequent increasing food demand determine an unprecedented need for agricultural products, which, in turn, drives deforestation, land degradation and overall loss of biodiversity ([61], [38]). This is even more evident when crop and livestock management is not sustainable and when the expansion of the agriculture frontier causes conflicts with detrimental effects on natural forests. The production of two commodities is pinpointed as the main driver of Amazon deforestation: beef cattle ([6]) and soybean cultivation ([39], [32], [49], [15]).
Sustainable agricultural practices are posited as the main alternative to counteract land degradation and deforestation while producing food resources. These practices in the Amazon include different types of agro-silvopastoral systems, agroforestry, crop rotation and pasture improvement ([16], [88], [78], [109]).
This paper has the overall objective to review the available literature on the adoption of sustainable practices (SP) in cattle ranching and soybean cultivation in the Amazon region. Initially, this will paint a broad picture of the diversity, typologies and geographical distribution of such practices and, then, enables a meta-analysis to calculate the effects of such practices on the ES. The aim is to increase the awareness of people and the scientific community about the existence of alternatives to the conventional and often unsustainable agricultural model, as developed in the last decades in the Amazon region, as well as to encourage the subsidizing of SP by public policies and initiatives. The above-mentioned need emerges as a response to climate change and biodiversity crises and, in parallel, the application of new pathways in agriculture and food systems are a stronger concern in the post Covid-19 scenario, where preventive sustainable approaches are expected to contribute to reducing the risk of new epidemic diseases ([30], [40]).
Materials and methods
This paper has been based on an extensive literature review followed by (i) a review analysis and (ii) a meta-analysis. The literature search was performed on indexed documents within the Scopus® platform based on a progressive combination of keywords in the string “Title, abstract and keywords”, then refined by “Keywords”, on 3 and 4 September 2020. The search included research articles, reviews, conference papers and book chapters published in English, French, Portuguese and Spanish from 1989 to 2020, that were focused on the Amazon region. The following keywords have been used and combined with the keyword “Amazon” or “Amazon region”: “sustainable cattle breeding”, “sustainable cattle farming”, “sustainable livestock”, “sustainable beef”, “integrated crop-livestock”, “sustainable intensification”, “silvopastoral systems”, “sustainable soy”, “sustainable soybean”, “soybean sustainability”, “soy zero deforestation”. The results of the first phase of literature search consisted of a large number of papers, so the second step of selection was done by filtering the papers according to the inclusion criteria of SP (sustainable management, silvopastoral systems and agroforestry) and sectoral policies (commodity chains and certification policies). The final database included 274 papers: 167 for cattle and 107 for soybean. We checked the full text of 88% of the available papers. The documents without available full text (the remaining 12%) were classified by using the abstracts so as to annotate full reference, year of publication, research location, and self-reported keywords (SRK from here on).
The review analysis was performed through three subsequent steps.
- Qualitative evaluation to discriminate the general characteristics of papers and their contents. The papers were grouped in 4 “topic” categories (TAA: Topic Assigned by the Authors): sustainable practices, sustainable management, certification policies, supply chain initiatives. We define as “sustainable practices” the concrete actions and experiments reported by the papers, while “sustainable management” refers to papers addressing general topics oriented to identify processes and procedures of managing more than the specific actions undertaken.
- SRK were extracted and those addressing the same concept were grouped to obtain a major conceptual understanding and to avoid excessive dispersion of terminology. We set a threshold of 6 keywords with converging meaning to generate a SKR group.
- The content of papers addressing SP for both drivers has been analyzed, with both quantitative and qualitative approach, in order to check the data suitability towards performing a meta-analysis.
The first search step for papers suitable for the meta-analysis resulted in 144 papers referring to the following topics: “integrated crop-livestock-forestry systems” (cattle), “livestock sustainable management” (cattle) and “integrated production systems” (soybean). The papers referring to the other topic categories (policies, supply chain initiatives) were excluded because the data reported were not consistent to apply the meta-analytic process. Afterward, we selected all papers related to the Amazon region (93 papers). The next selection excluded papers not including quantitative primary data (i.e., authors direct measurements in the field) so reducing the database to 40 papers. Later on, papers reporting extractable quantitative data suitable for performing the meta-analysis (mean values, standard deviation and sample size) were selected. The final selection consisted of 13 papers, comprising 6 SP (agropastoral, agrosilvicultural, agrosilvopastoral, crop rotation, pasture improvement and silvopastoral - see Tab. S1 in Supplementary material), whose ES were compared against conventional systems, defined and identified exclusively based on the information present in analyzed papers, with data usually shown as a specific column in a table. The studies usually refer to monoculture (e.g., soybean) or a pastureland without trees or crop rotation as a conventional system. Due to a high heterogeneity of the ES variables assessed in these studies, we reclassified the reported data according to 6 categories so as to allow a more robust comparison: animal productivity, herbage biomass, soil organic carbon (SOC), soil fertility, woody biomass and crop yield. Most studies compared more than one ES and more than one SP type. Furthermore, the timing of sampling (e.g., different years), the type of indicators, and the different depths in soil sampling are variables expressed by the datasets reported in the papers. We considered each comparison (i.e., a specific ES variable in a specific type of conventional system against a specific type of SP) inside the same study as an independent observation. As a complementary explanatory variable, we also extracted mean annual precipitation and temperature from the studies, and in the few cases in which they were not specified we used study location to get information from other published studies in the same area. Of these 13 papers, 7 presented data also comparing the SP systems against natural vegetation. The natural vegetation status or physiognomy definition varied across studies and was not always clear, but it comprehended mainly primary and late-successional forests (Tab. S1). With these 7 papers we performed a separate analysis to calculate the effect sizes of natural vegetation.
To investigate the effects of the adoption of SP on ES, the effect size was derived from the response ratio, obtained by subtracting the specific value of an ES variable (e.g., crop yield) in the reference system (e.g., monoculture cropland) from the value obtained in the SP, both log-transformed. This procedure is widely used in meta-analyses where the source data is heterogeneous ([113], [28]). Generally, a positive ratio indicates a positive effect of the SP, while negative values indicate negative influence. When this was not true for some specific variable, the signal was inverted to standardize the interpretation. Mean effect sizes, Z and p values and 95% confidence intervals were obtained by random effect models, and heterogeneity, both overall and within groups, was checked using a Q-test. We first performed an overall random effect model with all data, and then separated models for each variable group, SP type and their combinations. Study bias was checked with fail safe analysis, where a number larger than the number of studies indicates lack of bias. All analyses were performed using the “metafor” package in R ver. 3.6.1 ([100]).
Results
Results concerning the research location and the keywords analysis are presented first, for a better understanding. The results of the meta-analysis are presented immediately after, and in this case the evaluation has been performed jointly for the two drivers.
With regards to cattle ranching, 167 papers have been collected. Most of them were directly related to the Amazon region (70.7%), with a different distribution between countries (Fig. 1).
Fig. 1 - Number of livestock and proportion of papers in the Amazon region by country. Author’s elaboration based on FAOSTAT data (2019) and IBGE (Instituto Brasileiro de Geografia e Estatistica) - Censo Agropecuário 2017. Guyana, French Guyana and Suriname have not been considered for this figure, since data were not significant.
The other papers considered valid for the analysis (39.3%) regarded Latin American countries outside the Amazon basin and a global view about commodity chains and international policies and trade.
With regards to soybean, 107 papers have been collected. Most of them were directly related to the Amazon region (63.5%), predominantly in Brazil and to a lesser extent in Bolivia (Fig. 2).
Fig. 2 - Soybean harvested area (ha), production (tonnes) and proportion of papers in the Amazon region by country. Author’s elaboration based on FAOSTAT data (2019) and IBGE (Instituto Brasileiro de Geografia e Estatistica) - Censo Agropecuário 2017. Guyana, French Guyana and Suriname have not been considered for this figure, since data were not significant.
The other papers considered valid for the analysis (36.5%) regarded the Amazon region in a broader sense, mainly related to commodity chains and international policies and trade, and some examples of SP carried out in other Latin American regions, outside the Amazon basin.
In the selected studies about cattle ranching, the TAA with the highest number of studies was “livestock sustainable management” (46%), which includes a general approach to sustainability in the cattle sector, including the so called “sustainable intensification” (SI), followed by the topic “integrated crop-livestock-forestry systems” (28%), concerning mainly silvopastoral systems. Differently from cattle, the predominant categories of TAA for soybean regard papers related to policies (48%) and supply chain initiatives (39%). Papers dealing with sustainable agricultural practices represent only the 13% of the total, and mainly refer to crop rotation.
Regarding the most abundant SRK (N > 6) for cattle ranching, deforestation, silvopastoral systems and sustainability were the most cited, indicating that both the negative and positive aspects of cattle ranching and soybean cultivation were assessed in the studies (Fig. 3). For soybean, SRK referring to deforestation and land use change are largely predominant, and a lack of SRK addressing SP was noticed (Fig. 3).
Fig. 3 - Frequency of self-reported keywords (SRK) for cattle ranching (right) and soybean (left) studies.
In Tab. 1, a summary of SP for cattle ranching extracted from the analyzed papers, and their self-reported contributions to ES improvements are presented. The table is divided in two parts. The first part includes agroforestry, agrosilvopastoral and silvopastoral systems, which comprise a variety of combinations between shrubs and trees, forage plants (grasses and leguminous herbs) and livestock. All of these practices are indicated by the authors as strategies to achieve a more sustainable livestock production and to limit its pressure on tropical forests. The second part reports on the SI approach, intended as the implementation of practices oriented to increase crop and fodder production per area thanks to a more efficient use of inputs, so as to reduce pressure and impacts on land.
Tab. 1 - Sustainable practices in cattle-ranching and their role in improving ecosystem services. Qualitative aspect as self-reported by the authors of the reviewed papers or re-assigned during the analysis. (*): According to MEA ([80]): (S) Support; (P) Provisioning; (R) Regulating; (C) Cultural.
- | Proposed practice | Ecosystem services improvements*/ environmental positive effects (self-reported) |
References |
---|---|---|---|
Agroforestry, Agrosilvopastoral, Silvopastoral systems | Eucalyptus + soybean/corn intercropped with pasture in the interrow | S: increase of SOM; increased soil fertility and porosity; P: improved forage and meat yield; R: mitigation of tropical heat; C: alternatives to increase property revenues | Domiciano et al. ([31]), Magalhães et al. ([72]), Borges et al. ([9]), Moreira et al. ([84]) |
Eucalyptus, pine, teak, balsa + crops + livestock grazing |
S: increase of soil porosity | Oliveira et al. ([92]) | |
Trees, maize, grass, forage legumes and kudzu + natural forest regeneration + livestock grazing | S: enhanced species diversity; complete use of resources; pest protection; nutrients recycling; maintenance of soil structure; P: production diversification; C: adoptability for smallholders | Loker ([70]) | |
Trees (for timber, firewood and fruit) + forages + livestock grazing | S: diversity of tree species and forage species; multiple purpose of trees | Pizarro et al. ([98]) | |
Eucalyptus + livestock grazing (palisadegrass) | P: improved forage-based system; greater herbage accumulation; R: enhanced microclimate (mitigation); mitigation of cattle enteric emissions; increased soil C sequestration | Gomes et al. ([50]), Domiciano et al. ([31]), De Carvalho et al. ([23]), De Oliveira Resende et al. ([25]), Oliveira et al. ([93]) | |
African mahogany, eucalyptus and paricá (native species) + livestock | P: satisfactory tree performances; production diversification; C: alternatives to increase property revenues | Silva & Schwartz ([107]) | |
African mahogany and cumaru + livestock grazing | P: production diversification; R: increased soil C sequestration | Silva et al. ([108]) | |
Teak + livestock grazing | P: high quality products; production diversification; C credits; R: mitigation of erosion and desertification | Ansolin et al. ([3]) | |
ISPSs (Intensive Silvopastoral Systems) - high-density cultivation of fodder shrubs with improved tropical grasses and trees | S: enhanced biodiversity; soil conservation; P: production diversification; improved tree cover; R: climate change mitigation; increased soil C sequestration; water regulation; C: suitable for all farm scales, landscape restoration | Murgueitio et al. ([89]), Calle et al. ([11]), Murgueitio et al. ([88]) | |
Leucaena leucocephala + livestock grazing | S: enhanced biodiversity; N fixation (soil improvement); P: improved fodder resources; R: soil C sequestration; climate change mitigation | Chará et al. ([17]), Murgueitio et al. ([88]) | |
Mixed forage bank from different tree species | R: climate change mitigation; increased soil C sequestration; C: improved socio-economic conditions of farmers | Amézquita et al. ([1]) | |
Different tree species in rows + improved pasture with fodder bank | R: GHG emissions reduction; increased soil C sequestration | Landholm et al. ([62]) | |
Native trees and shrubs + rotational grazing systems | S: biodiversity conservation; R: water regulation, improved C stocks | Murgueitio et al. ([88]), Lerner et al. ([67]) | |
Sustainable Intensification | Crop-livestock integration: crop rotation (soybean/corn/cotton) + livestock grazing | S: soil conservation and improvement; P: higher annual net present value; R: C sequestration; reduction of soil greenhouse gases; lower GHG emissions; mitigation of climate change; | Soares et al. ([109]), Dos Reis et al. ([33]), Carvalho et al. ([13]), Gil et al. ([47]), Carvalho et al. ([12]) |
Rotational grazing | R: recovery of degraded pasture; low energy and water use; C: alternatives to increase property revenues | Pedrosa et al. ([94]), zu Ermgassen et al. ([117]), Gil et al. ([47]) | |
Pasture improvement (fertilization, irrigation, re-seeding) | S: increased pasture productivity; P: use of forage legumes; R: recovery of degraded pasture; C: low cost per ha | Pedrosa et al. ([94]), Latawiec et al. ([63]) | |
Introduction of forage legumes | S: soil conservation; soil properties improvement; functional biodiversity; R: water balance; mitigation of global warming and of groundwater contamination; rehabilitation of degraded land; C: saving of fossil energy | Schultze-Kraft et al. ([105]), zu Ermgassen et al. ([117]), Dubeux-Junior et al. ([35]), Hohnwald et al. ([57]) | |
Feedlot based intensification | R: decrease of on-property deforestation | Vale et al. ([114]) |
Meta-analysis on sustainable practices
From our initial database, only 13 publications comparing 6 types of SP against cropland and pasturelands met the requirements to be included in the meta-analysis, representing a total of 171 comparisons of ES variables between conventional systems and sustainable practices derived from the papers (hereafter SP1 dataset - Tab. 2). Although a minimum of 2 studies are recommended for a meta-analysis ([115]), we decided to include crop yield (only one study) due to its economical relevance in the Amazon, and also because it was evaluated in 3 different SP systems for a total of 35 comparisons. Six of the 13 publications also compared the SP against natural vegetation, for a total of 89 comparisons (hereafter SP2 dataset). In both SP1 and SP2 datasets most comparisons were made against silvopastoral systems (42.9% and 53.6%, respectively), and soil organic carbon (SOC) was the most representative variable assessed (28.6% and 55.1% of the comparisons, respectively).
Tab. 2 - Summary of the meta-analysis. Q represents the coefficient of the Q-test for heterogeneity and P its significance level. SP1 dataset included conventional cropland and pastureland as comparator; SP2 dataset included natural vegetation. (*): p < 0.05; (**): p <0.01; (***): p< 0.001; (ns): non significant.
Dataset | Moderator (Q, P) |
Ecosystem service | Effect size | SE | Z | p | 95% CI lower |
95% CI upper |
N |
---|---|---|---|---|---|---|---|---|---|
SP1 | Overall RE model | - | -0.025 | 0.017 | -1.429 | ns | -0.058 | 0.009 | 171 |
Ecosystem service (82.61; <0.001) |
Crop yield | -0.192 | 0.028 | -6.958 | *** | -0.246 | -0.138 | 35 | |
Herbage biomass | -0.152 | 0.03 | -5.101 | *** | -0.211 | -0.094 | 29 | ||
Livestock productivity | 0.194 | 0.096 | 2.027 | * | 0.006 | 0.381 | 9 | ||
SOC | 0.084 | 0.028 | 2.971 | ** | 0.029 | 0.14 | 49 | ||
Soil fertility | 0.029 | 0.032 | 0.922 | ns | -0.033 | 0.091 | 28 | ||
Woody biomass | 0.103 | 0.044 | 2.319 | * | 0.016 | 0.19 | 21 | ||
SP type (21.24; <0.001) |
Agropastoral | -0.088 | 0.048 | -1.822 | ns | -0.183 | 0.007 | 14 | |
Agrosilvicultural | -0.235 | 0.064 | -3.639 | *** | -0.361 | -0.108 | 13 | ||
Agrosilvopastoral | -0.085 | 0.046 | -1.855 | ns | -0.175 | 0.005 | 30 | ||
Crop rotation | 0.066 | 0.066 | 1.006 | ns | -0.063 | 0.195 | 9 | ||
Pasture improvement | 0.063 | 0.037 | 1.72 | ns | -0.009 | 0.135 | 32 | ||
Silvopastoral | -0.017 | 0.024 | -0.684 | ns | -0.064 | 0.031 | 73 | ||
SP2 | Overall RE model | - | 0.008 | 0.022 | 0.369 | ns | -0.036 | 0.052 | 89 |
Ecosystem service (2.39; 0.495) |
Herbage biomass | 0.019 | 0.225 | 0.086 | ns | -0.421 | 0.46 | 5 | |
SOC | 0.011 | 0.036 | 0.318 | ns | -0.058 | 0.081 | 49 | ||
Soil fertility | 0.049 | 0.027 | 1.784 | ns | -0.005 | 0.102 | 20 | ||
Woody biomass | -0.058 | 0.02 | -2.954 | ** | -0.096 | -0.019 | 15 | ||
SP type (0.75; 0.685) |
Crop rotation | -0.046 | 0.04 | -1.144 | ns | -0.124 | 0.033 | 9 | |
Pasture improvement | 0.014 | 0.052 | 0.272 | ns | -0.088 | 0.116 | 32 | ||
Silvopastoral | 0.013 | 0.022 | 0.587 | ns | -0.03 | 0.056 | 48 |
The random effect model including all studies in the SP1 dataset indicated a slightly negative, albeit non-significant, mean response ratio (RR) of the ES in the areas adopting SP (mean effect size = -0.024, p = 0.153 - Tab. 2, Fig. 4).
Fig. 4 - Mean effect size and confidence interval of SP compared to natural vegetation and cropland/pastureland. Mean effect size (dots) and 95% confidence interval (lines) of SP on the ES categories in comparison with conventional cropland and pastureland (upper panel) and natural vegetation (lower panel). 95% CI lines that do not overlap the dashed line are significant.
However, highly variable outcomes were found, as indicated by the significant heterogeneity index (Q = 9132, p < 0.001), although the fail safe analysis results showed no bias in the study (N = 26136). The overall random effect model in the SP2 dataset indicated a slightly positive, albeit non-significant, mean response ratio (RR) of the ES in the areas adopting SP (mean effect size = 0.008, p = 0.73 - Tab. 2, Fig. 4), also with high and significant heterogeneity (Q = 909, p < 0.001) but no sign of study bias (fail safe N = 1133).
Despite a positive trend, we found a marginal significant relationship between effect size and average annual rainfall (F = 3.50, p = 0.062) but no relationship with temperature (F = 0.33, p = 0.56; see Fig. S1 and Fig. S2 in the Supplementary material).
Comparison of ES and SP types
In the SP1 dataset, the mean effect size of the random effect models for the ES was positive (0.018), with SOC, livestock productivity and woody biomass positively affected by SP, while herbage biomass and crop yield were negatively influenced. The coefficient for soil fertility was also positive, although no significant differences were detected (Tab. 2, Fig. 4). In the SP2 dataset, woody biomass was negatively affected by SP, while no significant differences were found for the other variables (Tab. 2, Fig. 4). In the overall random effect models of the SP1 for each SP, only the agrosilvicultural model was significant, with negative effect sizes on the ES (Tab. 2, Fig. 4).
Comparison of ES by SP types
In the SP1 dataset random effect models testing the influences of SP on each ecosystem service, 7 combinations were significant. Among the significant combinations, crop yield was the only service negatively affected in all comparisons, while livestock productivity, SOC and woody biomass were positively affected in all comparisons (Tab. 3, Fig. 5).
Tab. 3 - Summary of the meta-analysis. (*): p < 0.05; (**): p <0.01; (***): p< 0.001; (ns): non significant.
Dataset | Sustainable practice |
Ecosystem service | Effect size | SE | Z | p | 95% CI lower |
95% CI upper |
N |
---|---|---|---|---|---|---|---|---|---|
SP1 | Agropastoral | Crop yield | -0.166 | 0.054 | -3.078 | ** | -0.271 | -0.06 | 8 |
Herbage biomass | 0.076 | 0.042 | 1.791 | ns | -0.007 | 0.159 | 3 | ||
Livestock productivity | 0.112 | 0.166 | 0.67 | ns | -0.215 | 0.438 | 3 | ||
Agrosilvocultural | Crop yield | -0.233 | 0.065 | -3.563 | *** | -0.361 | -0.105 | 9 | |
Soil fertility | -0.313 | 0.498 | -0.629 | ns | -1.288 | 0.662 | 4 | ||
Agrosilvopastoral | Crop yield | -0.178 | 0.035 | -5.063 | *** | -0.247 | -0.109 | 18 | |
Herbage biomass | -0.031 | 0.039 | -0.778 | ns | -0.108 | 0.047 | 3 | ||
Livestock productivity | 0.271 | 0.178 | 1.523 | ns | -0.078 | 0.619 | 3 | ||
Woody biomass | 0.14 | 0.148 | 0.945 | ns | -0.15 | 0.43 | 6 | ||
Crop rotation | SOC | 0.153 | 0.053 | 2.879 | ** | 0.049 | 0.257 | 6 | |
Soil fertility | -0.105 | 0.129 | -0.815 | ns | -0.357 | 0.147 | 3 | ||
Pasture improvement | SOC | 0.063 | 0.037 | 1.72 | ns | -0.009 | 0.135 | 32 | |
Silvopastoral | Herbage biomass | -0.195 | 0.029 | -6.643 | *** | -0.253 | -0.138 | 23 | |
Livestock productivity | 0.206 | 0.154 | 1.334 | ns | -0.097 | 0.508 | 3 | ||
SOC | 0.134 | 0.049 | 2.769 | ** | 0.039 | 0.23 | 11 | ||
Soil fertility | 0.055 | 0.029 | 1.903 | ns | -0.002 | 0.111 | 21 | ||
Woody biomass | 0.1 | 0.03 | 3.359 | *** | 0.041 | 0.158 | 15 | ||
SP2 | Crop rotation | SOC | -0.104 | 0.04 | -2.612 | ** | -0.182 | -0.026 | 6 |
Soil fertility | 0.067 | 0.041 | 1.629 | ns | -0.014 | 0.147 | 3 | ||
Pasture improvement | SOC | 0.014 | 0.052 | 0.272 | ns | -0.088 | 0.116 | 32 | |
Silvopastoral | Herbage biomass | 0.019 | 0.225 | 0.086 | ns | -0.421 | 0.46 | 5 | |
SOC | 0.066 | 0.039 | 1.698 | ns | -0.01 | 0.142 | 11 | ||
Soil fertility | 0.047 | 0.032 | 1.448 | ns | -0.017 | 0.11 | 17 | ||
Woody biomass | -0.058 | 0.02 | -2.954 | ** | -0.096 | -0.019 | 15 |
Fig. 5 - Mean effect size and confidence interval of SP in comparison with conventional cropland and pastureland. Mean effect size (dots) and 95% confidence interval (lines) of SP on the ES categories in comparison with conventional cropland and pastureland. 95% CI lines that do not overlap the dashed lines are significant.
Herbage biomass and soil fertility in turn were both positively and negatively affected. Regarding the SP types, only agrosilvicultural systems presented negative effects on the ES evaluated (crop yield and soil fertility). In the SP2 dataset, only 2 combinations were significant, SOC on crop rotation and woody biomass on silvopastoral systems, both with negative effect sizes. The other variables present in more than one SP presented both positive and negative effects (Tab. 3, Fig. 6).
Fig. 6 - Mean effect size and confidence interval of SP in comparison with natural vegetation. Mean effect size (dots) and 95% confidence interval (lines) of SP on the ES categories in comparison with natural vegetation. 95% CI lines that do not overlap the dashed line are significant.
Discussion
The review highlights that studies addressing SP in cattle ranching and soybean cultivation in the Amazon region are by far prevailing in Brazil (78.8% of total papers for cattle and 92.6% for soybean, respectively). This is firstly explained by the fact that almost 60% of the entire Amazon forest is located in Brazil. Secondly, the recent upturn in Amazon deforestation ([2]) has dramatically drawn attention again to the impact of permanent land cover changes and environmental degradation in the Brazilian Amazon. A third key factor is that Brazil is by far the largest soybean and livestock producer and trader among countries within the Amazon region ([18], [116]). Furthermore, as shown in Fig. 1and Fig. 2, the geographical distribution of studies reflects quite well how the two drivers are actually spread within the region. The only exception regards cattle ranching in Venezuela: according to FAOSTAT (Fig. 1), it has the third livestock number among Amazon countries, but, regrettably, no studies about this country were found in the literature.
Concerning the geographical distribution of SP, Brazil dominates, confirming trends. An interesting outcome of SP-country relations is that studies found in Brazil show a clear pattern in proposing practices which apply to the large-scale/industrial model, that characterize the Brazilian territory. In fact, Eucalyptus spp. or timber rentable species are dominant as tree species in agroforestry and silvopastoral systems, while the enhancement of tree species diversity appears rare in the proposed systems. This is in contrast with SP proposed for the other Amazonian countries. For Colombia, Peru, and Ecuador the proposed SP regarding diversified and complex silvopasture practices, which can be applied also at the small-scale, include native tree species and nitrogen-fixing plants, and consider a natural succession-based approach. Interestingly, self-reported ES do not differ so much between countries and type of SP. This suggests to take into consideration trade-offs among such diverse practices (e.g., the frequently cited C sequestration is often not correlated with biodiversity conservation).
SP in cattle ranching and soybean cultivation
The transformation of primary/secondary forest for agriculture, industrial legal/illegal logging, and pasture has been and is the main direct driver of tropical deforestation ([4]). Furthermore, a relevant part (the 50% of total pastures in Brazil, according to [29]) of Amazon pasturelands is classified as “degraded” and, consequently, shows a substantial decrease in productivity. SP for cattle ranching should address primarily the restoration of the productive potential of already existing degraded pastures ([16], [82], [88], [11], [83], [58], [62], [14]). In this perspective, Strassburg et al. ([111]) and Brandão et al. ([8]) estimated that increasing efficiency and productivity of Brazil’s grasslands and pasturelands could cover the current production of meat, crops, wood products and biofuels until 2040, without further conversion of natural ecosystems. As indicated by our review, different types of SP are available to contribute to pasture improvement and restoration.
The SI approach (Tab. 1) aims to achieve a best performing use of land in the livestock sector while restoring unproductive and degraded pastures. A first set of techniques includes rotational grazing ([47], [117], [94]), improved pasture diversification using forage legumes ([57], [35], [105], [117]), and crop rotation and crop-livestock integration ([45], [33], [109]). A second SI approach includes pasture improvement through fertilization, irrigation and/or re-seeding, cattle nutritional feed supplements, improved animal breeding, and feed-lot based intensification with confinement. The review revealed contradictions and trade-offs in SI application. The idea of SI proposes to produce more on increasingly smaller land. This could slow deforestation and free space for other food crops. However, controversial examples are reported: (i) the risk of “rebound effects”, related to intensification in agricultural frontiers, which can itself induce agricultural expansion by making the activity more rentable and attractive ([63], [117]); (ii) the connection between intensification and infrastructure development (road construction, slaughterhouses, etc.) which can increase deforestation ([63], [87]); (iii) the negative effects of confinement and feedlots on animal welfare and environment ([114]); (iv) the environmental trade-offs between intensified and extensive cattle production; (v) increasing demand for water associated with a growing cattle herd and additional resources for feed in feedlot finishing ([65]). Moreover, several practices behind SI can be capital intensive or require high use of resources and investment capacity, thus creating barriers and a possible divide between farmers, increasing leakage effects.
On the other hand, agroforestry, agrosilvopastoral and silvopastoral systems (Tab. 1) benefit from the functional and biological synergies of Soil-Plant-Animal-Atmosphere Metabolic Interactions (sensu [73]) well reported in the large mosaic of agrosilvopastoral solutions ([13]). Loker ([70]) proposed a model of a low external input agrosilvopastoral system for the tropics. This model includes well-adapted grass-legume pastures, rotational grazing and the management of natural forest regeneration. Hohnwald et al. ([58]) tested this model in practice and found that it represents a promising and ecologically sustainable alternative for smallholders. De facto, the importance of diversified farming systems incorporating farmer preferences and knowledge are currently increasing ([76]). Amézquita et al. ([1]), comparing different silvopastoral systems with native forest and degraded pasture, reported higher levels of SOC and concluded that they can constitute a viable economic alternative to farmers. Also, intensive silvopastoral systems, characterized by the high-density cultivation of fodder shrubs and trees combined with livestock grazing, are reported as successful alternatives for tropical countries ([89]). This approach proposes a “natural intensification” strategy ([11]), contributing to deconstruct the dichotomy between agricultural intensification and land sparing. The introduction of Leucaena leucocephala is referred as a useful practice ([17]). The inclusion of commercial timber species can constitute an interesting perspective for farmer income integration ([108], [3], [98]). The use of Eucalyptus spp. has a notable importance, mainly in recent papers referring to silvopastoral experimental practices in large properties ([23], [72], [31], [50]). Often, the use of Eucalyptus is related to the limitation of GHG emission intensity of cattle production ([54], [24], [26], [62], [36]), one of the reported benefits of silvopastoral systems.
Regarding SP and their adoption, though, cattle ranchers in the Amazon region generally show scarce interest. This lack of interest can be explained by the so called “Amazonian cattle culture” ([56]). Landowners choose to adopt more SP only when the marginal return of extensive ranching is lower than the intensifying one, and/or land becomes a scarce resource ([63]). Additionally, land speculation, which determines directly unproductive profit-seeking mechanisms, influences the low adoption of SP since the real objective is to maintain control over large areas while awaiting infrastructure development and higher land prices ([42], [104]). Other reasons that limit the adoption of SP include the lack of validation regarding economic viability ([92]), high implementation costs ([64], [68], [117]) - in a sector in which no-one is really willing to pay for improvements -, difficulties to access credits ([19]) and lack of incentives from public policies ([117]). However, the very limited adoption of sustainable agricultural practices is slowly changing, mainly because of the expected improvements in productivity and profitability, while the environmental concern is not a priority for cattle ranchers ([64]).
With regard to SP in soybean production, the overview differs from the performed analysis about cattle ranching. In fact, we have found a limited number of papers (13% - see Fig. 5) addressing sustainable soybean productive systems. Soybean is a large scale open-field crop, and this could explain the lower suitability in adopting integrated agriculture and/or agroforestry practices, perceived as small-scale practices. Consequently, some proposed options in this direction resulting from our review are: double-cropping systems ([53]); planting of soybean in crop-livestock integrated systems ([46]); and/or inclusion of tree species in integrated systems ([78]). These systems appear economically more sustainable ([33]), can contribute to mitigate GHG emissions ([12]) and to increase soil carbon stocks ([109]). Nevertheless, their success largely depends on successfully applied good management practices and technical support, and are still far from a broad application ([46], [93]). Furthermore, a reported factor of sustainability in soybean cultivation in the Amazon region is its establishment on previously cleared and/or abandoned pastures, addressing the potential restoration of degraded land. However, a strong side effect is the consequent displacement of cattle ranching to the forest frontier ([6], [32], [48], [74], [97]). In conclusion, as evidenced from the reviewed papers, it appears that the actual sustainability of soy refers essentially to public policies and supply chain initiatives that are supposed to reduce deforestation and mitigate soy negative impacts.
Meta-analysis of the effects of SP on ES
The first relevant finding of our meta-analysis was the low number of studies fitting the inclusion criteria, revealing a critical gap in the scientific knowledge of the use of SP in the Amazon. In a meta-analysis on the effects of agroforestry systems on biodiversity and ES in Europe ([113]), 53 studies were assessed, but with a clear geographical concentration, indicating that also in Europe there is an overall lack of empirical information.
Despite the relative low number of studies, the performed meta-analysis indicates that SP promoted an increase in four on six ESs as compared to conventional cropland and pastures and only woody biomass was lower as compared to natural vegetation. In the SP1 dataset, the two services that were negatively affected were crop yield and herbage biomass. Crop yield was negatively affected in all SP, and was responsible for the negative coefficient of the overall model. Such negative effect could be attributable to the shading effect of trees, and it was much higher in the corn crop (a C4 plant) than in soybeans (a C3 plant), the latter being significant only after the fourth year of SP implementation ([72]). The negative effects of SP on herbage biomass were somehow expected due to two major reasons: firstly, this variable was not measured in the pasture improvement studies; furthermore, as for crop yield, shading is known to reduce the productivity of grasses (C4 plants). However, studies indicating a negative effect on herbage biomass detected a positive and significant effect on livestock productivity. This result suggests that herbage biomass is not always a good predictor, as for example the thermal comfort (shade) provided by trees, or food production provided by woody species, may be relevant for livestock productivity ([59]). A similar trend was found in the European meta-analysis, with a negative relationship detected for biomass production, while food production was positively affected by agroforestry ([113]).
Soil organic carbon (SOC) was higher in all SP as compared to conventional cropland and pastures. In the silvopasture systems, the presence of trees directly improve the carbon translocation from the sub-soil, both as foliage decay and as deposition of woody material (e.g., branches). Additionally, trees increase soil humidity and protection from erosion, as well as enhance the abundance and diversity of soil organisms (fungi, bacteria, micro-arthropods), facilitate decomposition and, consequently, carbon accumulation ([20], [96]). Crop rotation and pasture improvement also enhanced the SOC content, indicating that even in the absence of trees these practices are beneficial for soil quality ([85]). In accordance with our findings, a meta-analysis of the effects of agroforestry on SOC stocks found positive effects for agroforestry systems in relation to conventional croplands, pasturelands and uncultivated areas in various soil horizons and depths ([28]). Nevertheless, these authors found that the conversion of forests to agroforestry decreased SOC amount, while in our case there was a positive, though non-significant, relationship. Such a result was an outcome of the comparison against pasture improvement systems, once well managed pasturelands are known to present high levels of SOC, although a relevant fraction was accumulated by the pre-existing forest before the pasture establishment ([85], [102]).
Soil fertility was positively affected by crop rotation, agrosilvicultural and silvopastoral practices in both SP1 and SP2 datasets. Due to a higher input of organic matter and a higher protection against environmental agents (rain, wind, sunlight), such practices enhance the accumulation and transformation of most nutrients ([58], [108]). The improvement of soil fertility and nutrient cycling in agroforestry systems has also been observed in Europe ([113]). In addition to the chemical components (nitrogen, phosphorous and sodium), our results indicated that SP practices also enhanced soil microbial and macro fauna abundance and diversity, a very important though often overlooked indicator of soil fertility ([7], [20]). Additionally, SP practices also reduced the potential of nitrification, a critical process in many agricultural fields ([21]).
As expected, woody biomass was higher in the silvopastoral and agrosilvopastoral systems evaluated. Besides producing important ES and improving biodiversity, woody biomass is an important source of income diversification ([3], [31], [107]), counterbalancing the reduction of crop yield and herbage biomass found in our results, although such direct comparisons are surprisingly poorly quantified in the literature ([113]).
Limitations of the meta-analysis
For a correct interpretation of our results, some considerations must be taken into account. The first limitation of our meta-analysis is the reduced number of studies meeting the selection criteria (only 13 out of 144). The studies were discarded because they lack primary quantitative data, comparisons against a reference system or required information (means, standard deviations and sample sizes), strongly reducing the spectrum of ES provided by SP in the Amazon. Such narrow filtering, however, is a common issue in meta-analysis, particularly those targeting complex agricultural practices such as agroforestry and variables related to ES or biodiversity ([99], [113], [28]). Another constraint for interpretation is the lack of standardization of the sampling protocols, as well as the high heterogeneity of the variables assessed, as mentioned in other meta-analysis ([28]).
Public policies and supply chain initiatives
The promotion of public policies, civil society actions and/or private arrangements on the meat and soy supply chain result as strategic assessments to mitigate the cattle and soybean sector’s negative impacts and to foster their sustainability. These initiatives include, on one side, government conservation interventions, such as incentive-based regulations, establishment of protected areas and expansion of indigenous lands, land regulations, and police operations against environmental crimes ([86], [66], [97]). On the other hand, interventions in the supply chain include the non-state market-driven governance systems ([10]), that should consider compulsory public-driven initiatives, policies and regulations and combine private sector, civil society and government interventions. They include roundtables, steering councils, zero deforestation agreements and other multi-stakeholder initiatives based on voluntary certification and market exclusion mechanisms, to create sustainable production standards ([27]).
With regards to cattle ranching, the TAA “certification policies” and “supply chain initiatives” were addressed by 26% of the papers reviewed (Fig. 2). Early measures to combat deforestation determined by cattle ranching (started in the early 2000s) were represented by public policies, primarily based on command-and-control instruments. Later on, pressure on the main meat distribution chains has increased, and this has determined the implementation of different initiatives. Representative examples, in the case of Brazil, are the TAC (literally: Term of Adjustment of Conduct) and the “zero-deforestation agreement”, signed in 2009. In both cases, meatpacking companies have committed to block acquisition from farms performing any deforestation after the agreement date ([44], [52]). Furthermore, the Brazilian Roundtable on Sustainable Livestock (GTPS), activated in 2009, based on a system of multi-stakeholder governance and claiming for a global/local large partnership, declares the commitment to zero deforestation, with the creation of the conditions and forms of compensation to make it viable ([27]). The stated goal of GTPS is to drive the transition towards the sustainability of the sector, thanks to certification standards, verifying that producers comply with established criteria and supporting firms to purchase certified products ([10]). A reported limit of these approaches is that they are hardly able to be widely applied, and generally they include only large-scale farmers/companies ([106]), reinforcing the cattle (and soybean) typical “productive exclusion” of small farmers ([79]). In fact, large farmers connected to global agribusiness are considered key players due to their scale of production; while in contrast, diversified systems of family farmers could have greater potential for sustainable development ([81]), but are hardly considered. Other limits reported are related to the fact that cattle are often raised on multiple properties prior to slaughter, fattened on noncompliant ranches, and then moved to a compliant property before sale to the slaughterhouses (so-called “laundering”), or even moved to regions not yet monitored by the agreements (so-called “leakage” - [44], [8]). This is mainly due to inadequate monitoring approaches and large segments of the cattle supply chain that are not tracked, meaning that such agreements do not necessarily translate into effective changes in sustainability ([10]).
Regarding soybean, many specific efforts to control deforestation emerged from the government, the private sector and the civil society. These topics are addressed by 87% of the collected papers (Fig. 5). In fact, at the global level, there is a growing recognition and awareness of the remote environmental damages driven by global food consumption, which encouraged many private- and public-sector commitments to reduce impacts ([51]). Besides the well-established effectiveness at reducing deforestation rates, the success of these interventions suffers numerous shortcomings. As an example, the Soy Moratorium, highly promoted in the last 15 years, represents the first large scale, voluntary zero-deforestation agreement created in collaboration between civil society, agribusiness industry and the Brazilian government, first signed in 2006 and extended to date. It is widely reported by the literature that, just after launching the moratorium, deforestation linked to soybean plantation in the Brazilian Amazon experienced a consistent decline ([103], [90], [5], [43], [60]). Nevertheless, the reduction of direct pressure of soybean on the Amazon forest indirectly displaced other activities, mainly cattle ranching, impacting again on the forest frontier ([74], [91], [97]). Furthermore, such reduction in the Amazon determined a displacement of soybean environmental pressure to other biomes, such as the Brazilian Cerrado (the current major soybean expansion frontier), concretely limiting the effectiveness of the moratorium ([34], [49], [101], [118]). Finally, most of the actual soy production is not monitored by the moratorium, making monitoring and cross checking impossible ([69]). Soterroni et al. ([110]) and Nepstad et al. ([91]) have proposed to expand the Soy Moratorium to the Brazilian Cerrado, taking into account that the Brazilian legislation is much less restrictive in terms of permitted clearings in this biome, compared to the norms applied in the Amazon. Another example of multi-stakeholder voluntary governance mechanism is the Roundtable on Responsible Soy, which creates certification standards to verify that producers comply with established criteria and to support firms purchasing certified products. Nevertheless, its actual extremely low adoption rate and the small area of application compared to global soy area is a limitation to its effectiveness ([41]). Furthermore, the soybean supply chain suffers from limited traceability. Leakage and laundering, processes through which soy grown on recently deforested area is included into the supply chain using tangled loopholes ([106], [118]), and noncompliance with legal requirements have been detected in properties that were “respecting” the moratorium criterion ([5]).
Conclusions
The results of the present review highlights a wide concern around the impacts of soybean crops and cattle ranching on the expansion of the agricultural frontier in the Amazon rainforest. The implementation of SP in their production chains is crucial. Nevertheless, research activities on indicators quantifying the benefits of applying SP need to be implemented, as highlighted by the meta-analysis. For example, despite the importance of crops in the Amazon region, also as deforestation drivers, crop-yield comparisons were found in only one study. This fact represents a very considerable gap and reflects a critical research weakness.
As a general conclusion, we should state that most of the papers are based on process- and discourses-oriented analysis more than deepening the robustness of claimed solutions. They draw a vivid picture of problems and statements, driving to governance approaches, but data on the effectiveness of SP, their comparability and replicability in research programs, are still very poor.
Nevertheless, some possible solutions stand out from our analysis. Regarding cattle ranching, it should be necessary to improve: the study and implementation of best practices; the incentive for increased productivity through technical assistance; and the decentralization of livestock keeping, instead of reinforcing the current trend toward larger landless livestock holdings. However, with regards to soybean cultivation, it seems that limited sustainable solutions, both for practices and policies, are available.
There remains an open question about how cattle ranching and soybean cultivation in the Amazon will become environmentally and socially sustainable in the long term. First of all, forests should no longer be reconverted to agricultural use. The reduction of soybean and cattle farming in the Amazon and the application of SP to increase efficiency and production of already existing productions appears to be crucial. The restoration and ecological improvement of degraded pastures, the use of agroforestry and silvopastoral systems should be supported, rather than industrial SI, whose numerous shortcomings have been demonstrated.
Profound changes in consumer behavior and diet are crucial, too: global reduction of meat consumption; fostering of food traceability; inclusion of the environmental costs of agricultural production in the price of food, to build a growing awareness among consumers; eating quality, not quantity. A comprehensive approach to achieving sustainability through a combination of solutions should be performed, to achieve social, economic and environmental objectives. In fact, the current focuses tend to ignore the negative externalities with respect to biodiversity conservation, social equality, land rights and climate change.
Furthermore, the pressure of deforestation in the Amazon might stimulate policies and their assessment should take into account the increasing coupling between production sectors and geographic locations and, at the same time, must be thoroughly grounded in an understanding of the region’s agriculture and social processes, including the land tenure issue.
A possible input for further research should be the broadening of research focus to other biomes under risk caused by the two drivers investigated in this study. In Latin America, this may be the case for the Chaco, a cross-country area (Argentina, Brazil, Bolivia and Paraguay) seriously threatened by degradation dynamics, or for the Brazilian Cerrado, highly endangered by soybean and cattle ranching expansion.
Acknowledgments
We gratefully thank the two anonymous reviewers for the complete and grounded comments and suggestions, which were very helpful to better define concepts and improve the robustness of our research. We also thank Andy Speak for his contribution to the English language revision.
References
CrossRef | Gscholar
CrossRef | Gscholar
CrossRef | Gscholar
CrossRef | Gscholar
Gscholar
CrossRef | Gscholar
CrossRef | Gscholar
Gscholar
Gscholar
CrossRef | Gscholar
CrossRef | Gscholar
CrossRef | Gscholar
Gscholar
Gscholar
Gscholar
Online | Gscholar
Online | Gscholar
CrossRef | Gscholar
CrossRef | Gscholar
CrossRef | Gscholar
CrossRef | Gscholar
CrossRef | Gscholar
Authors’ Info
Authors’ Affiliation
Department of Agriculture, Food and Forest Sciences (SAAF), University of Palermo (Italy)
Claudia Cocozza 0000-0002-0167-8863
Fabio Salbitano 0000-0002-3592-9632
Department of Agriculture, Food, Environment and Forestry (DAGRI), University of Florence (Italy)
Lab. of Global Ecology, Department of Biosciences and Territory, University of Molise, Pesche, IS (Italy)
Corresponding author
Paper Info
Citation
da Silveira Bueno R, Marchetti L, Cocozza C, Marchetti M, Salbitano F (2021). Could cattle ranching and soybean cultivation be sustainable? A systematic review and a meta-analysis for the Amazon. iForest 14: 285-298. - doi: 10.3832/ifor3779-014
Academic Editor
Marco Borghetti
Paper history
Received: Feb 09, 2021
Accepted: Apr 12, 2021
First online: Jun 08, 2021
Publication Date: Jun 30, 2021
Publication Time: 1.90 months
Copyright Information
© SISEF - The Italian Society of Silviculture and Forest Ecology 2021
Open Access
This article is distributed under the terms of the Creative Commons Attribution-Non Commercial 4.0 International (https://creativecommons.org/licenses/by-nc/4.0/), which permits unrestricted use, distribution, and reproduction in any medium, provided you give appropriate credit to the original author(s) and the source, provide a link to the Creative Commons license, and indicate if changes were made.
Web Metrics
Breakdown by View Type
Article Usage
Total Article Views: 5120
(from publication date up to now)
Breakdown by View Type
HTML Page Views: 2047
Abstract Page Views: 336
PDF Downloads: 2360
Citation/Reference Downloads: 0
XML Downloads: 377
Web Metrics
Days since publication: 1145
Overall contacts: 5120
Avg. contacts per week: 31.30
Article Citations
Article citations are based on data periodically collected from the Clarivate Web of Science web site
(last update: Nov 2020)
(No citations were found up to date. Please come back later)
Publication Metrics
by Dimensions ©
Articles citing this article
List of the papers citing this article based on CrossRef Cited-by.
Related Contents
iForest Similar Articles
Research Articles
Assessing food sustainable intensification potential of agroforestry using a carbon balance method
vol. 12, pp. 85-91 (online: 24 January 2019)
Research Articles
A Decision Support System for trade-off analysis and dynamic evaluation of forest ecosystem services
vol. 11, pp. 171-180 (online: 19 February 2018)
Review Papers
Towards better practices of salvage logging for reducing the ecosystem impacts in Mediterranean burned forests
vol. 13, pp. 360-368 (online: 25 August 2020)
Review Papers
Payment for forest environmental services: a meta-analysis of successful elements
vol. 6, pp. 141-149 (online: 08 April 2013)
Review Papers
Paying for water-related forest services: a survey on Italian payment mechanisms
vol. 5, pp. 210-215 (online: 12 August 2012)
Short Communications
Competition effects and economic scenarios in an agroforestry system with cereal crops and wood plantations: a case study in the Po Valley (Italy)
vol. 14, pp. 421-425 (online: 15 September 2021)
Research Articles
Green oriented urban development for urban ecosystem services provision in a medium sized city in southern Italy
vol. 7, pp. 385-395 (online: 19 May 2014)
Review Papers
Shaping the multifunctional tree: the use of Salicaceae in environmental restoration
vol. 6, pp. 37-47 (online: 21 January 2013)
Research Articles
Vascular plants diversity in short rotation coppices: a reliable source of ecosystem services or farmland dead loss?
vol. 13, pp. 345-350 (online: 17 August 2020)
Research Articles
Payments for forest environmental services: organisational models and related experiences in Italy
vol. 2, pp. 133-139 (online: 30 July 2009)
iForest Database Search
Search By Author
Search By Keyword
Google Scholar Search
Citing Articles
Search By Author
Search By Keywords
PubMed Search
Search By Author
Search By Keyword