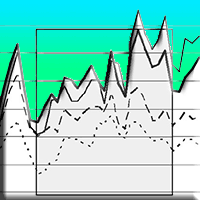
Scots pine’s capacity to adapt to climate change in hemi-boreal forests in relation to dominating tree increment and site condition
iForest - Biogeosciences and Forestry, Volume 14, Issue 5, Pages 473-482 (2021)
doi: https://doi.org/10.3832/ifor3703-014
Published: Oct 18, 2021 - Copyright © 2021 SISEF
Research Articles
Abstract
Forest site (FS) and meteorological conditions are recognized as the main factors affecting tree growth and whole-stand sustainability. This study aims to detect the combined effects of FS and meteorological conditions on tree ring formation of Scots pine (Pinus sylvestris L.), the most common tree species in Lithuania and hemi-boreal forests of northeastern Europe. We used data on stand structure and productivity from the Lithuanian National Forest Inventory (NFI) and stem radial increment series of dominating trees during the period 1993-2012 collected since 2013. Pine stem basal area increment (BAI) was chosen as the response variable, while temperature in March (°C) and precipitation in June (mm) were used as predictor variables, as they best express the effect of climate change on Lithuanian forests. We simulated the effects on dominating pine annual increment of deciduous tree species, mainly Betula sp. and the level of soil moisture and fertility, accounting in addition for the random effects of NFI network tract, plot direction, and tree number. A nonlinear mixed-effects model explained up to 68% of the variation in the BAI of pine trees. The annual pine trees BAI increased with the increase in the proportion of deciduous trees in pine stands. Increases in temperature and precipitation in considered months reinforced this positive effect on pine BAI, especially in mature pine stands in temporarily waterlogged meso-eutrophic FSs on mineral soils. A negative effect of deciduous trees on pine stem increment was observed only in nutrient-rich eutrophic and drained peatland FSs. Forestry treatments directed towards the increase in deciduous tree proportion in the most common normal or temporarily waterlogged meso-eutrophic and oligotrophic pine stands might increase the biodiversity and productivity of pine stands, and their sustainability in future climate change scenarios.
Keywords
Scots Pine, Basal Area Increment, Site Conditions, Meteorology, Mixed-effects, Hemi-Boreal Forests
Introduction
There is great concern worldwide about the effects of climate change on forest growth, because forests account for a large portion of the sequestered carbon by terrestrial ecosystems. Climate change is expected to increasingly impact forest ecosystems ([8]), as it could affect forest area, health and biodiversity. On one hand, climate change may increase growth rates in temperate and boreal forests ([36]), while on the other hand, temperature-induced drought stress can endanger the survival of trees and forest communities ([46], [64], [49]), especially in southernmost areas ([55]). In northeastern europe, such changes are expected to have a positive influence on boreal forest growth with an increase in productivity. However, the impact of climate warming in the hemi-boreal zone, which is the transition zone between boreal and temperate zones, depends on site conditions ([34]). Such divergent forest productivity trends challenge our capability to effect projections of tree growth dynamics under the pressures of climate change ([41]).
The impact of climate change on productivity varies according to geographic area, species, stand composition, tree age, soil fertility and water holding capacity, as well as the interactions of these parameters ([35], [53], [41]). Therefore, forest site-specific conditions like soil moisture and fertility can be key factors limiting tree growth ([15]). However, our understanding of the effects of climate on forest health, productivity, and the carbon cycle is limited for many regions and ecosystems, especially under different or opposite site conditions ([62], [40], [10]). Usually, such observations are based on small sample sizes, which makes it difficult to extrapolate them to the regional or national forest scale ([59]). The influence of species mixing on the reaction of pine to environmental change needs more detailed investigation, especially in different site conditions ([1], [10]).
Tree responses to climate change have been analyzed in relation to soil conditions ([53]). Tree rings are widely used to study the impact of climate change on forest carbon cycling, and for validating process-based models of forest productivity where temporal variation are determined by large trees ([65]). Understanding the effects of climate change on forest productivity and its time series is critical, and tree rings offer an annually resolved proxy to explore tree growth over times when no forest inventories or meteorological instrumental records were available ([11]). Tree-ring series are widely used to reconstruct stand productivity history. Therefore, the impact of climate change on forest tree growth, as well as past growth trends ([32], [43]) have been extensively analyzed through series of tree rings ([19], [17]), especially of dominating trees in forest stands ([65]).
Scots pine (Pinus sylvestris L.) was chosen in this study as the main species to detect the effect of increased temperature and precipitation on tree annual increment. It is one of the most common tree species not only in Lithuania but also in the entire hemi-boreal and boreal european region ([48]). Our earlier findings in northeastern Lithuania revealed that since 1980 Scots pine trees showed significant increases in annual basal area increment (BAI). This was related to significant long-term increases in air temperature in September, early spring and July. Heat and drought in June limited pine tree growth, but only on organic soils under excess-moisture regime, which increased the sensitivity of pine to drought ([2], [4], [5]).
In northeastern europe, air temperature and precipitation increased over the 20th century and particularly since the late 1980s ([21], [5], [41]). Recently Lithuania became a top hotspot in Europe. There greatest shifts in thermal and precipitation regimes have been detected, with mean temperature increasing by +0.325 °C and precipitation by +20 mm per decade ([56]). The adaptation capacity of pine forests to such climate changes is one of the major challenges for forest management in this region. It is necessary to understand the effects of climate change on pine growth to be able to predict how these effects might affect pine stand sustainability in hemi-boreal forests in the future. Recently, the proportion of deciduous tree, especially Betula spp. in pine stands, has also been shown to have a major effect on pine tree annual increment in relation to tree age, density and site condition ([1]). In this study we attempted to determine and assess: (i) the effects of key meteorological parameters on pine tree annual BAI in forest sites with different levels of soil moisture and soil fertility; (ii) the effect of the proportion of deciduous trees in pine stands on pine annual BAI under different soil conditions; (iii): the effect of tree age on pine tree annual BAI under different forest site conditions in relation to selected meteorological parameters; (iv) future changes in pine tree annual BAI in hemi-boreal pine forests under forecasted climatic conditions.
The results could suggest new forestry treatments ensuring sustainable forest development under the pressures of global change in hemi-boreal forests.
Materials and methods
National Forest Inventory data
Data on stand, tree and soil characteristics were collected on the NFI plots during the fourth National Forest Inventory (NFI) 5-year cycle from 2013 to 2017 (Fig. 1). Scots pine cores were collected at breast height from one selected dominating tree of each tree species growing in the same stand, but outside of the permanently monitored plot. Annual increment of the dominating tree of each tree species in a stand was selected as the main response variable to detect the integrated effects of environmental predictor variables. The sample size for dendrochronological analysis during the entire 5-year cycle consisted of 2414 Scots pine trees over 40 years old from the same number of sample plots. More than 2500 cores were taken from other tree species.
Fig. 1 - Location of Scots pine sample plots and structure of NFI permanent plots at 4 km distance from each other across Lithuania. The 250 × 250 m tract with four 500 m2 circle plots at its different directions represents a plot.
We eliminated data on pine BAI from stands younger than 40 years to reduce variability in tree-ring width data, as the variability in younger stands is high due to tree competition and tree density ([27], [57], [24], [25], [13]).
Dendrometric parameters of trees were obtained from four circle plots (500 m2 in size and 12.76 m in radius) in each NFI tract, which were located on the tops of isosceles triangles at 4 km distance from each other both horizontally and vertically (Fig. 1). The identification of a tract and the position of a plot in the tract (direction), as well as the tree identification number (id) were used to account for random effects on pine BAI formation in mixed-effects models. Forest type and soil conditions were also assessed in the plots using the Lithuanian NFI methodology ([29]).
BAI calculation of sample dominating trees
We measured annual tree-ring width in stem cores, from bark to pith, with an accuracy of 0.01 mm, using an electronic transducer and a binocular scope fixed over the moving stage of the Lintab™ v.6 tree-ring measuring equipment, and TSAP-Win™ software (RinnTech e.K., Heidelberg, Germany). Individual tree-ring width series were synchronized by a visual comparison of graphs for each ring width series with averaged pine tree ring width series of the correct age group ([14]). We calculated the Pearson’s correlation coefficients and means among and between each tree ring width series for the right age group ([6]). This procedure ensured significant differences in annual increments among years, but synchronic and non-significant differences in the rhythm of growth in relation to tree diameter at different ages ([49]).
The data on tree ring widths over the last 5-year period (2013-2017) were not included in the analysis to avoid not fully formed increments of the last year to have equal period for each tree.
To detect recent regular pine tree growth, the period between 1993 and 2012 was chosen for analysis (Fig. 2). This 20-year period when mean temperature and precipitation increased by 0.049 °C and 1.92 mm per year, respectively, ([5]) reflects climate change quite well. This increase is statistically significant and is in full agreement with the SReS A1 B Project ([21]).
Fig. 2 - Annual values of precipitation and temperature in Lithuania with added trends for the considered 20-year period (left) and effect of their monthly values on changes in mean BAI values of all pine trees during the 20-year period (right).
Basal area increment (BAI) series were derived from tree ring width series. BAI was used instead of ring width, due to its higher relationships with stem volume increment and lower interrelationship with tree diameter and tree ageing process, as well as recent tree age. This parameter captures growth trends better than tree-ring width and avoids the need for data detrending ([7]). Such detrending could also remove low frequency variability that could hide the effects of meteorological parameters with the same variability.
Meteorological data
Meteorological data were collected from 16 meteorological stations located across Lithuania. Mean monthly precipitation and temperature were used in the BAI analysis. Mean temperature in March and precipitation in June were chosen as they have the most significant effect on the pine increment not only in Lithuanian forests (Fig. 2) but also in boreal and hemi-boreal forests ([58]). This is in agreement with our previous results ([25], [26], [3], [4], [5]). Climatic parameters were assigned for each tree using an inverse distance weighted (IDW) interpolation method based on the distance to meteorological stations located nearby ([12]).
Forest sites
Scots pine responses to the considered meteorological parameters were analyzed based on growing conditions defined by a classification of stands into forest site types. These types describe edaphic and hydrological site conditions and are the main classification units used in Lithuanian forestry. In the present study, four forest sites (FS) differing in soil moisture and four FSs differing in soil fertility level were defined. Moisture regime of the FSs, defined in this study as “Water” independent variables, varied from normal moisture (W1) and temporary over moisture (W2) to drained (W3) and undrained peatland (W4). FS fertility level, defined in this study as “Nutrients” independent variables, varied from very oligotrophic (N4) and oligotrophic soils (N3) to mesoeutrophic (N2) and eutrophic soils (N1). This characterization of the monitored forest sites allowed us to detect the effects of soil properties on pine growth.
Scots pine BAI modeling
The linear mixed-effects (LMe) model from the R package “NLMe” ([42]) was used to analyze differences in annual BAI under different soil conditions. Tract id, plot position in the tract, and tree id were included as random effects in all the models. An asterisk (*) in the model indicates interactions between the main group and the remaining three “Water” or “Nutrients” groups. The predict function in the R package “stats” v. 3.6.0 was used to display the model values with average group parameters.
BAI data logarithms (log) were used to remove heteroscedasticity from the model residuals and after the simulation were back-transformed to tree BAI using exponent function. Biological plausibility of the results was used to evaluate the model ([9]). All the statistical analyses were carried out using R software ([50]).
The BAI model in relation to the moisture regime of the FS referred to as “Water” is described as follows (Water model - eqn. 1):
where BAI is the basal area increment in year t. Fixed effects are composed of four soil moisture regime groups (Water: W1, W2, W3, W4); T(III) is the mean temperature in March; P(VI) is the sum of precipitations during June; Mix is the proportion of pine trees in the mixed pine stand, where 1 indicates a pure pine stand; and BA is the tree basal area in year t-1, calculated from ring width.
An interaction comparison was performed on normal moisture sites (W1), which were the most common with N = 1682 trees. Where p<0.05 indicated significant differences between the effect of predicting variables on pine BAI in the main forest site and the rest FSs, while p>0.05 indicated no statistical differences of this effect, i.e., effect of predicting variables was the same like in the main selected forest site.
BAI model in relation to soil fertility level of FS is described as follows (Nutrient model - eqn. 2):
where BAI is the basal area increment in year t. Fixed effects are composed of four “Nutrients” groups based on soil fertility level (Nutrients: N1, N2, N3, N4); T(III) is the mean temperature in March; P(VI) is the sum of precipitation during June; Mix is the proportion of pine trees in the mixed pine stand; BA is the tree basal area in year t-1, calculated from ring width.
Interactions between BAI and predict variables were compared to the mesotrophic site (N2) as this FS is not only quite represented in our sample, but is also the FS where the most productive pure pine stands could be developed in hemi-boreal coniferous forest.
In the present study, the effect of deciduous trees in pine stands is expressed as the proportion of pine trees in the pine stand (“Mix”), defined as (eqn. 3):
where DBA is the basal area of deciduous trees in a pine stand; WSBA is the whole stand basal area.
To evaluate the effect of tree age on the relationships between pine BAI formation and meteorological variables in different mixed pine stands, data obtained in normal humid (W1), temporary waterlogged (W2), mesotrophic (N2), and oligotrophic (N3) soil FSs were used. Very oligotrophic (N4) FS stands were excluded from this analysis due to the inadequacy of oligotrophic soils for the growth of deciduous trees. Three age groups were selected, each age group containing more than 200 samples of pine BAI series from the same pine stands. BAI model in relation to the selected tree age, referred to as “Age”, is described as follows (Age model - eqn. 4):
where the fixed effects are composed of Age, classified into three age groups (60-, 80-, and 110-year-old); T and P parameters, which are as defined above for the “Nutrients” and “Water” models. An interaction comparison was performed on the youngest 60-year age group.
Determination coefficients (R2) were used to evaluate the models. Marginal R2 describes the proportion of variance explained by the fixed factors alone; conditional R2 describes the proportion of variance explained by the fixed and random factors ([39]). The effects of fixed factors in the BAI model were evaluated using the level of significance (p). Model estimate values were back-transformed using the “exp” function in R.
Results
Overall data evaluation
The sampled trees for BAI estimation were distributed in different FS groups based on “Nutrients” and “Water” levels. The regular FS condition of pine stands in Lithuania is normal humid (W1) and oligotrophic soil (N3). These groups were the most represented in the study. Representation of the other FSs was lower (Tab. 1). Pine tree stands from drained peatland (W3) and eutrophic soil (N1) FSs were least represented in the study, because these FSs are less represented overall for pine growth in Lithuania. The most productive FSs were on temporally waterlogged soils (W2), and mesoeutrophic soils FS (N2).
Tab. 1 - Mean stand characteristics of FSs in soil water and nutrients groups, and their core samples. (BAI): annual basal area increment for a 20 year period from 1993 to 2012 (cm2); (Age): stand age (year); (Mix): mean proportion of pine in the mixed pine-deciduous stand; (Dbhplot): mean diameter of trees at breast height (1.3 m) at a sample plot (cm); (Hplot): mean height of trees at a sample plot (m); (Age_plot): age of the stand (years); (Cores): number of sampled trees selected for BAI estimation (units). Dbh and Age are the main characteristics of the sampled pine trees selected for BAI estimation. (*): Mean characteristics of pine stands obtained from NFI data base; (**): Mean characteristics from dominant in stand trees selected for BAI estimation.
Data set |
Mean | Unit | Water | Nutrients | ||||||
---|---|---|---|---|---|---|---|---|---|---|
W1 | W2 | W3 | W4 | N1 | N2 | N3 | N4 | |||
NFI* | Dbhplot | cm | 28.9 | 29.2 | 24.4 | 14.8 | 29.4 | 30.2 | 28.1 | 21.3 |
Hplot | m | 25.9 | 26.2 | 23.1 | 14.1 | 26.1 | 27.1 | 25.4 | 18.5 | |
Mix | - | 0.95 | 0.88 | 0.84 | 0.91 | 0.80 | 0.88 | 0.94 | 0.97 | |
Age | yrs | 85 | 85 | 90 | 88 | 83 | 81 | 87 | 85 | |
BAI estimates** |
BAI | cm2 | 10.36 | 10.98 | 9.12 | 4.91 | 12.38 | 12.21 | 9.54 | 7.71 |
Dbh | cm | 32.3 | 34.0 | 29.1 | 19.3 | 37.0 | 35.3 | 31.4 | 24.9 | |
Age | yrs | 77 | 78 | 81 | 87 | 74 | 74 | 80 | 77 | |
Cores | n | 1682 | 286 | 139 | 157 | 37 | 471 | 1464 | 292 |
BAI data reflect the productivity of the separate FS groups. The least productive pine stands grow on undrained peatland (W4) and very oligotrophic (N4) soils. Pine growth at these sites is extremely limited by an over-moisture regime and lack of nutrients compared to the other FSs. Only a few sampled pine trees represented eutrophic soil (N1) FSs, as these sites are mainly occupied by deciduous tree species and the pines growing there tend to form big crowns with large branches that ruin the overall wood quality and do not form pure stands. This FS is not typical of pine growth in a hemi-boreal forest.
Results from the ANOVA two-factor analysis of BAI variation indicated significant differences among the years over the considered 20-year period, as well as among four moisture and fertility FS groups (Fig. 3).
Fig. 3 - Annual basal area increment (BAI) of the sampled dominating pine trees in pine stands of different moisture and fertility levels. Shaded area indicates the 20-year period of investigation.
Effect of meteorology on pine BAI for different “Water” FS groups
The results showed that temperature in March had a significant effect on pine BAI variation at W1 forest sites (Tab. 2 - Water model, MT row). March temperature also had a positive effect on the other FSs. An increase in temperature by 1 °C resulted in an increase of pine BAI in the different “Water” FS groups of 0.19 cm2 for W1, 0.26 cm2 for W2, 0.20 cm2 for W3, and 0.07 cm2 for W4 (Fig. S1 in Supplementary material). The only significant difference was found between the effect of temperature on BAI in temporarily waterlogged (W2) vs. normal moisture (W1) FSs (Tab. 2 - W2:MT, p < 0.001).
Tab. 2 - Models’ parameters and significance values of predicting variables. NFI tract, direction, and tree id were used as random effects. (MT): mean temperature in March; (JP): precipitation amount in June; (SD): standard deviation of the random effects. For more details on parameters, see text.
Model | Fixed effects | |||||
---|---|---|---|---|---|---|
Parameter | Estimate | Confidence interval (95%) | Std. Error | p-value | ||
lower | upper | |||||
Water model | Intercept | -0.124 | 0.112 | 0.137 | 1.053 | <0.001 |
W2 | -0.919 | 0.834 | 1.013 | 1.051 | 0.089 | |
W3 | 1.134 | 0.976 | 1.317 | 1.079 | 0.101 | |
W4 | -0.71 | 0.62 | 0.815 | 1.072 | <0.001 | |
MT | 1.022 | 1.021 | 1.023 | 1.001 | <0.001 | |
JP | 1 | 1 | 1 | 1 | <0.001 | |
log(Mix) | -0.701 | 0.55 | 0.896 | 1.133 | 0.005 | |
log(BA) | 3.687 | 3.586 | 3.791 | 1.014 | <0.001 | |
W2:MT | 1.007 | 1.004 | 1.01 | 1.002 | <0.001 | |
W3:MT | 1.004 | 1 | 1.009 | 1.002 | 0.071 | |
W4:MT | -0.996 | 0.992 | 1 | 1.002 | 0.069 | |
W2:JP | 1 | 1 | 1 | 1 | 0.186 | |
W3:JP | -0.999 | 0.999 | 1 | 1 | 0.003 | |
W4:JP | -0.999 | 1 | 1 | 1 | 0.769 | |
W2:log(Mix) | -0.819 | 0.525 | 1.279 | 1.254 | 0.381 | |
W3:log(Mix) | 2.851 | 1.577 | 5.153 | 1.352 | 0.001 | |
W4:log(Mix) | -0.62 | 0.264 | 1.457 | 1.545 | 0.273 | |
Nutrients model | Intercept | -0.106 | 0.095 | 0.119 | 1.122 | <0.001 |
N1 | 1.29 | 0.947 | 1.757 | 1.171 | 0.106 | |
N3 | -0.999 | 0.921 | 1.084 | 1.043 | 0.99 | |
N4 | -0.986 | 0.882 | 1.102 | 1.059 | 0.803 | |
MT | 1.019 | 1.016 | 1.021 | 1.001 | <0.001 | |
JP | 1.001 | 1 | 1.001 | 1 | <0.001 | |
log(Mix) | -0.694 | 0.513 | 0.938 | 1.166 | 0.018 | |
log(BA) | 3.84 | 3.736 | 3.948 | 1.034 | <0.001 | |
N1:MT | 1.003 | 0.995 | 1.012 | 1.004 | 0.568 | |
N3:MT | 1.005 | 1.003 | 1.008 | 1.001 | <0.001 | |
N4:MT | 1.003 | 0.999 | 1.007 | 1.002 | 0.114 | |
N1:JP | -0.999 | 0.999 | 1.001 | 1 | 0.592 | |
N3:JP | -0.999 | 0.999 | 1 | 1 | <0.001 | |
N4:JP | -0.999 | 1 | 1.001 | 1 | 0.002 | |
N1:log(Mix) | 2.509 | 0.924 | 6.812 | 1.665 | 0.072 | |
N3:log(Mix) | 1.245 | 0.839 | 1.847 | 1.223 | 0.277 | |
N4:log(Mix) | 1.343 | 0.562 | 3.212 | 1.56 | 0.507 | |
Age model | Intercept | -0.088 | 0.071 | 0.11 | 1.238 | <0.001 |
80 | -0.964 | 0.848 | 1.097 | 1.068 | 0.582 | |
110 | -0.841 | 0.737 | 0.96 | 1.07 | 0.011 | |
MT | 1.018 | 1.014 | 1.022 | 1.002 | <0.001 | |
JP | 1.001 | 1 | 1.001 | 1 | <0.001 | |
log(Mix) | -0.565 | 0.359 | 0.889 | 1.257 | 0.014 | |
log(BA) | 4.109 | 3.885 | 4.345 | 1.068 | <0.001 | |
80:MT | 1.003 | 0.998 | 1.008 | 1.003 | 0.297 | |
110:MT | 1.005 | 1 | 1.011 | 1.003 | 0.041 | |
80:JP | -0.999 | 1 | 1 | 1 | 0.85 | |
110:JP | -0.999 | 0.999 | 1 | 1 | 0.229 | |
80:log(Mix) | 1.486 | 0.824 | 2.681 | 1.352 | 0.189 | |
110:log(Mix) | -0.934 | 0.504 | 1.733 | 1.371 | 0.831 | |
Model | - | Random effects standard deviation | Determination coeff. R2 | |||
- | Tract id | Direction | Tree id | Marginal | Conditional | |
Nutrients model | - | 1.261 | 1.238 | 1.395 | 0.29 | 0.658 |
Water model | - | 1.243 | 1.23 | 1.396 | 0.313 | 0.662 |
Age model | - | 1.325 | 1.205 | 1.419 | 0.25 | 0.644 |
Pine BAI in drained peatlands (W3) and natural peatlands (W4) differed also from the normal moisture sites (W1) when the effect of temperature on BAI formation (Tab. 2) was accounted for, but only close to the level of significance (p < 0.1 - Fig. S1 in Supplementary material).
The effect of June precipitation on pine BAI formation at W1 FS was also highly significant (Tab. 2 - Water model, JP row), but less significant than the effect of March temperature in other FSs. In W1, W2 and W4 FSs, a 10 mm increase in precipitation increased BAI by 0.16 cm2, 0.32 cm2 and 0.05 cm2, respectively. Precipitation in drained peatland soils (W3) had a negative effect on the BAI of -0.26 cm2 per 10 mm, significantly different from the effect of precipitation on BAI in the normal moisture regime W1 (Tab. 2 - W3:JP, p < 0.003). For the other FSs, the effect of precipitation on BAI was positive, and did not differ significantly from the effect of precipitation in normal soil moisture sites W1 (W2:JP, p > 0.18) and especially in peatland soils (W4:JP, p > 0.76).
The results on the “Intercept estimate” for W2, W3 and W4 compared to group W1 when the effect of the other variables in the model were eliminated (equal to 0) shows that BAI in the W1 FS differed quite significantly from the other groups (Tab. 2 - lines W2-W4, p < 0.01). The highest level of significance was between BAI in the W1 and W4 FSs (p < 0.05). Thus, the soil moisture regime modifies significantly the effect of the meteorological parameters on pine BAI. The integrated effect of meteorological and stand mixture parameters increased the significance among the BAI in different moisture FSs, explaining more than 66% of the variation.
Effect of meteorological conditions on pine BAI in different “Nutrients” FS groups
Both meteorological parameters also had a highly significant positive effect on pine BAI in main mesotrophic (N2) FS. An increase in March temperature by 1 °C resulted in an increase in pine BAI in all groups of “Nutrients” FSs, i.e., by 0.24 cm2 in N1, 0.19 cm2 in N2, 0.20 cm2 in N3, and 0.13 cm2 in N4 (Fig. S2 in Supplementary material). The only significant difference was found between the effect of temperature on BAI in oligotrophic soils N3 and in mesotrophic soil N2 (Tab. 2 - N3:MT, p < 0.001). Quite different effect of temperature on BAI was found in very oligotrophic soils (N4) if compared with data obtained in (N2). Difference was close to the level of significance (N4:MT, p > 0.1). No significant difference was found between the effect of temperature on BAI between eutrophic N1 (N1:MT, p > 0.5) and mesoeutrophic soils N2. This means that March temperature had a similar significant effect on pine BAI at both eutrophic (N1) and mesoeutrophic soils (N2) FSs.
Similar results were obtained for the effects of June precipitation on BAI at FSs with different nutrient levels. An increase in precipitation by 10 mm in June resulted in an increase in pine BAI in all groups of “Nutrients” FSs, i.e., by 0.36 cm2 for N1, 0.53 cm2 for N2, 0.06 cm2 for N3, and 0.03 cm2 for N4 (Fig. S2 in Supplementary material). No statistical difference was detected in the effect of precipitation on BAI between eutrophic N1 (N1:JP, p > 0.5) and mesoeutrophic soil N2. The effect of precipitation on BAI of pine trees growing in oligotrophic soils (N3) and very oligotrophic soils (N4) FSs significantly differed from the effect of precipitation on BAI at mesotrophic (N2) FSs (Tab. 2 - N3:JP and N4:JP, p < 0.001) and also at eutrophic N1 FSs.
The results on the “Intercept estimate” for N1, N3 and N4 compared to the FS N2 value when the effect of the other variables in model were eliminated (equal to 0) revealed that differences in BAI were not statistically significant among “Nutrients model” groups (Tab. 2 - lines N1-N4, p > 0.05). This is in full agreement with the fact that Scots pine has lower demand for nutrients than for water. Further, significant differences in BAI in different nutrient soil conditions were found, mainly depending on age and interactions with meteorological and stand mixture parameters explaining up to 65% in BAI variation. The comparison between “water” and “nutrients” effects revealed that soil moisture was more important for pine BAI than soil nutrients if other parameters are constant.
Effect of tree age on relationships between pine BAI formation and meteorology in different FS groups
Both meteorological parameters had a significant and positive effect on the BAI of pine trees of ages varying between 40 to 70 years. Tree age had a significant effect on the relationship between temperature and BAI (Fig. S3 in Supplementary material). An increase in temperature by 1 °C resulted in an increase in pine BAI by 0.16 cm2 for 60 year old trees, 0.19 cm2 for 80 year old trees, and 0.23 cm2 for 110 year old trees (Fig. S2). There were no significant differences in the effect of temperature on the BAI of 70 to 90 year old trees, however, the effect on the BAI of trees over 100 years old was significant (Tab. 2 - 110:MT, p < 0.04). The increase in temperature resulted in more intensive increase in BAIs for mature or over-mature pine trees than for younger trees. It is the key finding of the effect of climate warming on pine BAI in hemi-boreal forests.
The effect of precipitation on BAI was also found to be positive, but tree age had no significant effect on these relationships (Fig. S3 in Supplementary material). Precipitation had the most effect on pine BAI in stand ages between 60 and 110 years, but this difference was not statistically significant (110:JP, p > 0.2). We conclude that both temperature and precipitation had a positive effect on pine BAI, the effect varied with age classes, and in all cases, temperature had a stronger effect than precipitation.
The results on the intercept estimated for the 80- and 110-year age groups compared to the 60 year group when the effect of other variables in model were eliminated (equal to 0) revealed that only differences between BAI from the first and third age group were significant. The obtained differences in BAI were found mainly depending on age and interactions, with meteorological and stand mixture parameters explaining up to 64% of BAI variation.
Effect of deciduous tree proportion in pine stands in different “Water” and “Nutrients” soil FSs and age groups
In this study the increase in deciduous tree proportion (DTP) in mixed pine stands (decrease in the “Mix” parameter, i.e., pine proportion in pine stand) also had a positive effect on BAI formation, but only in typical Scots pine FSs. The negative effect of deciduous trees was found only in forest stands on drained peatland soils W3. For normal moisture FSs (W1), a 10% reduction in pine proportion (increase in deciduous trees proportion) increased pine BAI by more than 0.5 cm2, while in drained peatland (W3) soil FSs decreased by almost 0.7 cm2 (W3:log(mix) - Tab. 2, Fig. S4). The relationships between other FSs (W2 and W4) showed a similar positive increase in BAI when the pine mixing proportion decreased.
The relationship between BAI formation and proportion of pine in stands at mesotrophic soil (N2) FSs did not differ significantly from the relationships established in the other “Nutrients” FS groups, with the exception of the eutrophic N1 FS, where the effect of stand mixture on BAI was close to the level of significance (p < 0.07). There, a 10% decrease in pine proportion (increase in deciduous tree proportion) resulted in pine BAI increase by 0.63 cm2 in mesoeutrophic soil (N2) while in eutrophic soils (N1) in BAI decrease by almost -0.9 cm2. At oligotrophic N3 and very oligotrophic N4, a 10% increase in deciduous trees proportion in pine stands increased pine BAI by 0.2 cm2 and 0.07 cm2, respectively. This effect of deciduous trees on pine BAI did not differ significantly from that detected in mesoeutrophic N2 FSs.
As the FS fertility decreased from mesotrophic to very oligotrophic soils, there was a clear decrease in the positive effect of deciduous trees on pine BAI. Pine trees growing in stands with a higher deciduous tree proportion (Fig. S4 in Supplementary material) had a higher BAI than pine trees growing in pure pine stands, especially at N2 FSs. The opposite effect on pine BAI was observed in eutrophic soils (N1). There, deciduous trees inhibited pine BAI formation. This site is not typical for Scots pine in hemi-boreal forests.
Tree age had no significant effect on the relationship between pine BAI and deciduous tree proportion in mixed pine stands. A 10% reduction in pine proportion in a stand (or increase in deciduous tree proportion) resulted in pine BAI increase from by approximately 0.9 cm2 in younger pine (60) up to 1.1 cm2 in older pine (110).
An increase in the proportion of deciduous trees resulted in both increase/decrease of pine BAI more significantly at FSs under different moisture regimes vs. different levels of fertility (Fig. 4). A 10% increase in DTP resulted in an increase in pine BAI by approximately 9.0% at W4, a little lower at W2 and the lowest 4.6% at W1, while by only 4.8% at N2, a little lower at N3 and the lowest, only 1.0%, at N4 FS. Age of pine stand had no significant effect on the increase in pine BAI with increasing DTP in mixed stands and made about 7-8% in middle aged and mature stands.
Fig. 4 - Comparison of the effect of the 10% and 50% ratio of deciduous trees in pine stands at different FSs under recent climate changes on annual increments of a single dominating tree in pine stands.
The most significant effect of deciduous trees on pine BAI was observed at N2 and W4 FSs. The positive effect of deciduous trees at N3 and W1 FSs, which dominate in Lithuania, was slightly lower. A negative effect of deciduous trees resulting in the reduction of pine BAI was established only at N1 and W3 FSs, not typical for pine growth in Lithuania.
Fig. S4 (Supplementary material) shows that a DTP up to approximately 50% in mesoeutrophic soil N2 FS resulted in the same pine BAI as in pure pine stands in eutrophic soils FS (N1). The basal area increment of pine trees was around 13 cm2 per year. On the other hand, DTP of up to 50%, which increased pine BAI in oligotrophic (poor) fertility soils (N3) by up to 9 cm2 per year, reduced pine BAI by a similar level in eutrophic soils (N1).
Such opposite effects of the proportion of deciduous tree species, mainly Betula spp., on pine growth should be taken into account in silvicultural measures for sustainable development of boreal or hemi-boreal coniferous forest.
Discussion
Main methodological approach of the study
Forest inventory data collected based on rigorous statistical methods provide reliable data on forest productivity since the circle plots are the same size in all sampled areas and are evenly located, forming a tight network in the area of investigation. Caution should be exerted, however, with the use of such datasets to detect the effects of meteorology or new meteorological threats on pine boreal forest. Tree ring width data series are the most reliable as the accuracy of annual tree ring width data obtained from tree stem analysis is typically higher than DBH data obtained from permanent sample plots of national forest inventories ([18]). Short time series and poor age records are mentioned among the most concerning problems, together with the fact that dendrometric data are often recorded with much lower precision ([63]).
Tree rings are also widely used to understand the impact of climate change on forest carbon cycling, and to validate process-based models of forest productivity, where temporal variation of productivity is determined by large trees ([65]). Combining conventional forest inventory data and annual increment data based on increment cores of dominant trees in stands offers great potential to evaluate growth patterns under different forest conditions in relation to environmental factors. In this study, an evaluation of the capacity of Scots pine to adapt to climate change was undertaken on the basis of Lithuanian National forest inventory (NFI) data on stand structure and productivity, supplemented with tree ring width series of single dominating pine trees. This methodological approach allowed to establish the main tendencies in BAI formation of pine trees under different FS conditions and to detect key meteorological parameters, which affect pine forests in Lithuania.
Radial growth of trees within forest stands greatly depends on the interactions between competition and environmental conditions ([44]). According to the current state of knowledge, dominating tree parameters reflect well the productivity of a fully stocked even-aged stand because they are independent of density, or competition intensity ([60]), and therefore, are used as a measure of site productivity ([38]). Parameters of dominating trees are the key parameters in site index models ([45], [52]). They are also often included in forest development models used for the estimation of species mixing effects or of the effect of forestry measures, expressed through variation in stem radial growth ([63], [59]).
It is essential to carefully consider tree sample design in dendrochronological analyses, in order to estimate precisely tree increment, their changes, and the effects of climate change, minimizing the sampling effort from more than 2000 permanent observation plots of Lithuanian national forest inventory net. We sampled from 1 up to 2-3 tree cores per plot to ensure reliable representation of mixed pine stand productivity and productivity variations during the last few decades, even though only one tree ring series per tree species was represented. The results confirmed our hypothesis on the relationship between the mean values of pine tree BAI (based on dendrochronological data of dominating single pine trees) and gross stand volume increment (based on NFI data). The Wilcoxon test for all species including Scots pine supported the null hypothesis (p = 0.650-0.972), meaning that there was no significant difference in paired series gathered using different methodologies, i.e., between the data collected within the frame of NFI and the data collected from tree-level dendrochronological investigation ([28]). A regression model created with the NFI data on stand volume increment as the dependent variable and tree-level BAI as the independent variable was characterized by significant coefficients of determination exceeding 70%.
To meet the objectives, W1 and N2 FSs were used as references for water and nutrient levels in forest stands as they were considered to represent best growing conditions for Scots pine in Lithuania. Every factor was compared against this reference to evaluate differences in chosen factor groups (soil moisture or nutrition). Such comparisons help to find factors that positively or negatively affect BAI at given soil moisture or nutrient levels.
To reduce BAI variation among the stands, standwise calibration was performed based on tree BA. It is known that BAI captures growth trends better than tree-ring width and avoids the need for data detrending ([7]). However, in our model, significant relationships between BA and BAI remained, indicating direct effects of stem diameter and tree age on BAI. This relationship could be attributed to the effect of climate warming, when the observed increase in temperature had a more significant effect on the increase in BAI of the older and bigger pine trees than on the younger and thinner trees.
Much of data variability resided in the random effects. Differences between corresponding marginal R2 (variance explained by fixed factors) and conditional R2 (variance explained by fixed and random factors) reflect how much variability is related to random effects (Tab. 2). Unstructured random components (tree and plot location together with tree id) were included into the models to account for subject-specific BAI variations through the nonlinear mixed-effects modeling approach. In the present study the introduction of these components helped to explain differences between different growth conditions that could not be accounted for otherwise. Such differences within or between plots of the same soil type may be due factors we did not account for in the model such as genetics or competition. Recently, this approach has been used to increase the prediction accuracy of forest growth models ([16], [59]). In such situations it is claimed that a mixed-effects modeling approach is the most appropriate, as it takes into account the hierarchical data structure and includes all the subject-specific variations, stochasticity, and randomness present in the data ([10]). Inclusion of the parameters of dominating trees (BA) and pine stands (mixture, site condition, age) together with subject-specific random effects into the BAI models significantly increased the explanation of BAI variation and the biological importance of the models especially in stands of pine mixture with deciduous tree species, mainly Betula spp.
Effect of deciduous tree proportion (DTP) on pine stem BAI
Forest mixtures offer great potential for silviculture, not only due to the higher resilience and resistance to various biotic and abiotic climate-related disturbances but also due to higher productivity and capacity to mitigate risks associated with climate change ([48], [8]). However, their growth dynamics are often difficult to predict because spe-cies interactions vary with climatic and edaphic conditions, stand structure and forest management ([1]).
DTP in pine stands had a beneficial effect that could be explained by better light distribution in the tree canopy and higher nutrient level in the upper soil horizon due to deciduous leaf litterfall. This result agrees well with findings on the beneficial effects of deciduous trees in pine stands, evidenced as increased adaptability of mixed stands to recent changes ([31]), and in some cases, increased productivity when compared to similar mono-species stands ([54], [22]).
Soil properties might affect the interaction between deciduous and pine trees. Jactel et al. ([22]) reported that the relationship between biodiversity and productivity can become more positive or more negative under different soil conditions, especially with limited water or nutrient supply ([51], [23]). As availability of water increases, the availability of nutrients decreases, resulting in an increase in light competition ([47]).
Maestre & Cortina ([33]) also claimed that when a limited resource is the only fundamental abiotic stress factor, facilitation could only occur when deciduous trees increased the availability of this resource for the coniferous tree species. Such interactions might possibly be happening in the poorest peatland soils FSs, i.e., at combined W4 and N3 and W4 and N4 FSs, which could explain the increased pine growth at such sites when useful minerals are most likely scarce (oligotrophic FS). These relationships confirm results on very nutrient reach N1 and water regime interrupted drained W3 FS where deciduous trees reduced pine BAI.
The results on the mixture effect in pine forests revealed that the highest magnitude of changes in pine BAI was obtained applying the “Water” model (eqn. 1) and confirmed the statement that availability of water is a key parameter of pine productivity in hemi-boreal forest in northeastern europe ([47], [22]).
Our results on the benefits of mixtures of pine and deciduous trees contribute to the knowledge that carefully designed mixed Scots pine stands could bring a wider range of ecosystem services and be more resilient than pure stands, while also increasing productivity ([22], [54], [47], [48]).
Effect of meteorology on pine stem BAI
Scots pine growth is affected by frost, heat and drought throughout Europe ([1]). The radial growth responses of the eastern Baltic population of Scots pine showed explicit regional gradients depending on local climates, indicating gradual shifts in the effects of winter temperature and summer moisture regimes ([34]).
The effect of June precipitation on BAI at FSs with different moisture regimes was not as significant as expected. Despite its positive and significant effect on BAI in almost all FSs, a negative effect of precipitation in drained peatland soil (W3) was detected with a slight decrease in BAI. At this FS, a decrease in BAI with increasing proportion of deciduous trees in pine stands was observed, possibly due to light competition with faster growing deciduous trees like ash, alder or birch. Trees with lower average DBH, but similar height were observed at the drained sites when compared to the other sites except for the peatland sites (W4 - Tab. 1). Similar results were obtained by Hökkä & Groot ([20]) with drained sites, as they found that growth might be limited by excess soil water in low stock stands that possibly had damaged drainage systems. An annual report of the Lithuanian Mineral and Mining Sector ([61]) stated that 54% of drained sites are in bad working condition and 15% have insufficient drainage, mainly due to activity of ineffectively managed beaver communities. It is possible that the effect of higher precipitation in beaver-damaged stands resulted in episodes of waterlogged regime causing a stress reaction in pine trees.
On the other hand, changes in tree growth at northern latitudes is mainly limited by the availability of water in sandy soils ([37]), making tree growth there more sensitive to climatic variation ([30]). Possibly, the effect of precipitation during June on BAI formation at FSs with lower water availability differed significantly in comparison with the effect observed at waterlogged soil FSs.
Our findings showed that the effect of precipitation in June on pine BAI was more significant than the effect of temperature in March in different nutrient levels, while the effect of temperature in March was more significant than the effect of precipitation in June in FSs with different moisture levels. These findings agreed with our earlier results showing negative effects of drought and positive effects of climate warming on pine basal area increment in locally or regionally polluted forest ([24], [25], [26], [4], [5]). No negative effect of increased temperature on pine BAI in the remaining months was established. Therefore increases in early spring temperature and precipitation amount during vegetation could promote Scots pine tree growth and overall yield of pine stands in Lithuania.
Conclusions
From our study we conclude that:
- an increase in mean temperature in March resulted in an increase in dominating pine tree BAI more significant than an increase in precipitation amount in the middle of the vegetation period, when intensity of this increase in older pine stand was higher than in younger ones; it is the key finding of the effect of climate warming on pine BAI in hemi-boreal forests;
- an increase in the proportion of deciduous trees in mixed pine stands had a positive effect on dominating pine tree BAI in typical for Scots pine growth FSs and most importantly in oligotrophic soil (N3) prevailing in Lithuania;
- in drained peatland soils (W3), an increase in precipitation during June and of the deciduous tree ratio in pine stands had a negative effect on dominating pine tree BAI; the same negative tendency was observed in the stands on eutrophic soil (N1);
- tree age had a significant effect only on relationships between dominating pine tree BAI and mean temperature in March; the effect of age on the relationships between pine tree BAI formation and precipitation amount was not significant;
- forestry treatments directed toward the increase of deciduous tree proportion in the most common normal or temporarily waterlogged meso-eutrophic and oligotrophic pine stands might increase not only the biodiversity of pine stands, but also their productivity, and could help the hemi-boreal pine forest adapt to and mitigate the new threats of climate warming.
Acknowledgments
We would like to thank Dr. Peter Biber from the Munich Technical University for helping with the statistical analysis. This research was supported by the Forest Policy Group of the Ministry of Environment of the Republic of Lithuania.
References
CrossRef | Gscholar
Gscholar
CrossRef | Gscholar
CrossRef | Gscholar
Gscholar
Gscholar
Gscholar
CrossRef | Gscholar
CrossRef | Gscholar
CrossRef | Gscholar
Authors’ Info
Authors’ Affiliation
Gintautas Mozgeris 0000-0002-8480-6006
Edgaras Linkevičius
Ingrida Augustaitiene
Algirdas Augustaitis 0000-0003-3938-3611
Vytautas Magnus University, K. Donelaičio str. 58, LT-44248 Kaunas (Lithuania)
Technische Universität München, Am Hochenger, D-85354 Freising (Germany)
Corresponding author
Paper Info
Citation
Mikalajunas M, Pretzsch H, Mozgeris G, Linkevičius E, Augustaitiene I, Augustaitis A (2021). Scots pine’s capacity to adapt to climate change in hemi-boreal forests in relation to dominating tree increment and site condition. iForest 14: 473-482. - doi: 10.3832/ifor3703-014
Academic Editor
Gianluca Piovesan
Paper history
Received: Nov 20, 2020
Accepted: Aug 17, 2021
First online: Oct 18, 2021
Publication Date: Oct 31, 2021
Publication Time: 2.07 months
Copyright Information
© SISEF - The Italian Society of Silviculture and Forest Ecology 2021
Open Access
This article is distributed under the terms of the Creative Commons Attribution-Non Commercial 4.0 International (https://creativecommons.org/licenses/by-nc/4.0/), which permits unrestricted use, distribution, and reproduction in any medium, provided you give appropriate credit to the original author(s) and the source, provide a link to the Creative Commons license, and indicate if changes were made.
Web Metrics
Breakdown by View Type
Article Usage
Total Article Views: 1350
(from publication date up to now)
Breakdown by View Type
HTML Page Views: 0
Abstract Page Views: 0
PDF Downloads: 1068
Citation/Reference Downloads: 3
XML Downloads: 279
Web Metrics
Days since publication: 1013
Overall contacts: 1350
Avg. contacts per week: 9.33
Article Citations
Article citations are based on data periodically collected from the Clarivate Web of Science web site
(last update: Nov 2020)
(No citations were found up to date. Please come back later)
Publication Metrics
by Dimensions ©
Articles citing this article
List of the papers citing this article based on CrossRef Cited-by.
Related Contents
iForest Similar Articles
Research Articles
Growth patterns of Scots pine (Pinus sylvestris L.) under the current regional pollution load in Lithuania
vol. 8, pp. 509-516 (online: 12 November 2014)
Research Articles
Coping with spring frost-effects on polyamine metabolism of Scots pine seedlings
vol. 10, pp. 227-236 (online: 27 January 2017)
Research Articles
Modeling compatible taper and stem volume of pure Scots pine stands in Northeastern Turkey
vol. 16, pp. 38-46 (online: 22 January 2023)
Research Articles
Twenty years of conversion: from Scots pine plantations to oak dominated multifunctional forests
vol. 10, pp. 75-82 (online: 19 October 2016)
Research Articles
Effects of planted European beech on the understory in Scots pine forests of Lithuania
vol. 7, pp. 12-18 (online: 07 October 2013)
Technical Advances
Improved estimates of per-plot basal area from angle count inventories
vol. 7, pp. 178-185 (online: 17 February 2014)
Research Articles
A comparison of models for quantifying growth and standing carbon in UK Scots pine forests
vol. 8, pp. 596-605 (online: 02 February 2015)
Research Articles
The growth dynamics of East European Scots pine (Pinus sylvestris L.) populations - a Lithuanian field trial
vol. 17, pp. 59-68 (online: 06 March 2024)
Research Articles
Effects of mixture and management on growth dynamics and responses to climate of Quercus robur L. in a restored opencast lignite mine
vol. 15, pp. 391-400 (online: 05 October 2022)
Research Articles
A fast screening approach for genetic tolerance to air pollution in Scots pine field tests
vol. 6, pp. 262-267 (online: 01 July 2013)
iForest Database Search
Search By Author
Search By Keyword
Google Scholar Search
Citing Articles
Search By Author
Search By Keywords
PubMed Search
Search By Author
Search By Keyword