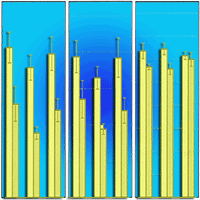
Effects on soil characteristics by different management regimes with root sucker generated hybrid aspen (Populus tremula L. × P. tremuloides Michx.) on abandoned agricultural land
iForest - Biogeosciences and Forestry, Volume 11, Issue 5, Pages 619-627 (2018)
doi: https://doi.org/10.3832/ifor2853-011
Published: Oct 04, 2018 - Copyright © 2018 SISEF
Research Articles
Abstract
Fast-growing Populus species are becoming frequently used at afforestation of arable land globally and hybrid aspen is promising for short rotation forestry in the northern hemisphere. Knowledge about growth performance of the second-generation plantations, i.e., consisting of root sucker generated shoots after clearcutting of the original stand, is increasing, but less information is available on the effects on soil properties, especially with varying management. We followed the soil effects of three different management regimes, including 4-, 8- and 16-year rotations with thinning measures in the two longer rotations, in root sucker generated hybrid aspen on former agricultural land. The study was performed in a randomized block design and changes in soil variables were estimated by repeated sampling, i.e., at root sucker initiation and after eight years. Concentrations and pools of soil organic carbon (SOC) and soil nutrients, pH and bulk density were analysed in the 0-15 and 15-30 cm mineral soil. Common for all management regimes were unchanged SOC and nutrient pools; pH and bulk density were also unaffected during the study period. Afforestation effects on the vertical distribution of nutrients, i.e., redistribution of NH4-N, K and Mg from deeper to shallower soil by plant uptake and release through litter decomposition, were observed in all management regimes. A different effect was noted for NO3-N where a declining trend was observed. This could indicate a leakage of the ion, but NO3-N distributions in soils are variable which makes interpretations difficult. The results suggest that different management strategies have small initial effects on mineral soil characteristics. However, soil changes need to be followed for an extended period to get more information on the long-term impact of afforestation and management of root sucker generated stands.
Keywords
Afforestation, Bulk Density, Nutrient Removal, pH, Rotation Time, Soil Organic Carbon (SOC), Soil Nutrients
Introduction
The genus Populus includes several fast-growing tree species that are easily cultivated on both forest and abandoned agricultural land. Poplar plantations cover an area of 8.7 billion ha globally ([4]) and are mainly used for bioenergy, pulp and log production, but also for environmental purposes such as soil protection, phytoremediation and mitigation of high atmospheric CO2 levels by carbon (C) sequestration in biomass and soil. Their natural habitat covers the entire northern hemisphere and species like European aspen and North American trembling aspen (P. tremula L. and P. tremuloides Michx.) are among the most widespread tree species globally ([37]). Hybrid aspen is a crossing between those two species and has mainly been bred in Sweden, Finland and Germany ([37]). The hybrid has shown a high potential for biomass production under north European conditions on former agricultural land, reaching 8-10 Mg DM ha-1 year-1, which is more than most other tree species at northern latitudes ([45], [18], [38]). Both parent species and their crossing generate dense root sucker stands after clear cutting, i.e., a second generation. Hybrid aspen may produce 50.000-100.000 shoots ha-1 from root suckers in this second generation ([24], [27]). Thus, the ability for root sucker sprouting in combination with the high production potential makes hybrid aspen promising for short rotation forestry in the Nordic region. Short rotation forestry with hybrid aspen is a relatively new silvicultural approach in this region and further research is needed on various management, physiological and environmental aspects concerning not only the first-generation plantation, but also the second generation emerging from root suckers ([46]).
Hybrid aspen is considered a suitable tree species for afforestation of abandoned agricultural land. Growing aspen and poplars on fertile arable sites are known to promote high growth rates ([46]) and there may be a significant potential for mitigating high atmospheric CO2 levels by sequestering C in biomass and soil ([40]). Arable soils contain 50-75% of their natural (i.e., before the conversion to arable land) C stocks in general and thus have a capacity to sequester C to recover their original levels ([23]). Site preparation, planting procedure and mechanical weed control can lead to soil disturbance with subsequent initial release of CO2. Studies of afforestation with Populus species suggest that soils at first act as sources and later as sinks for C ([13], [12], [20], [5]). But there are also studies where no decrease in soil organic carbon (SOC) were observed initially ([42]). Short-rotation forestry implies frequent harvesting intervals and removal of large quantities of biomass including nutrients ([36]). It is therefore crucial to monitor changes in pH and plant nutrients, to detect eventual nutrient deficiency or risk of leakage. So far, studies of soil effects at afforestation with hybrid aspen have dealt with first-generation plantations ([25], [26], [42]). Those studies found no changes in soil organic carbon (SOC) or total nitrogen (Tot N) in the upper 30 cm soil 5-15 years after planting, but observed an increase of K and Mg in the top 10 cm soil and a decrease below, indicating a redistribution of those base cations from deeper soil layers to the upper soil ([42]), and decreases in CN ratios and pH over time ([26], [42]).
Effects of forest management on SOC and Tot N are important to understand from the aspects of soil fertility and C storing capability. Harvesting removes biomass, disturbs the soil and changes the microclimate which may lead to increased C losses in the soil ([16]). However, a meta-analysis found that forest harvesting on average had little or no effect on soil C or N, except for conifers where saw log harvesting caused increases and whole tree harvesting decreases ([19]). Thinning measures result in similar primary effects as harvesting, i.e., soil disturbance and altered microclimate, but less experimental knowledge on the effects of thinning on soil properties is available ([16]). Most of the literature on harvesting and thinning effects on soil characteristics to date refers to conifers. A study of spruce and pine on podsol found that the effects of repeated thinning on soil chemistry (pH, C, N, P and base cations) were variable but generally small ([34]).
Studies on growth performance and effects of management on the next generation of hybrid aspen plantations, i.e., shoots generated from root suckers after clear cutting of the original stand, have recently been initiated in Sweden ([27]). An evaluation of productivity and sustainability of second-generation hybrid aspen subjected to different management regimes with respect to length of rotation period and extent of thinning measures has been made eight years after initiation ([39]). The management regimes included 4-, 8- and 16-year rotations with thinning measures in the two longer rotations. The 16-year rotation had not yet reached full time and was evaluated midterm. All regimes included removal of biomass after harvest or thinning measures, except for a latter thinning in the 16-year rotation. A simultaneous study of the effects of those management regimes on soil characteristics was performed and is reported here. We wanted to test the hypotheses that: (1) mineral soil characteristics, i.e., concentrations and pools of SOC, Tot N, base cations and pH, would be significantly influenced by management regime; and (2) changes in soil characteristics could be reflected in the amount and timing of removed biomass, including nutrients, at harvest and thinning operations.
Materials and methods
Site description
The study was performed in root sucker plots of hybrid aspen (Populus tremula L. × P. tremuloides Michx.). The site is situated in southern Sweden at latitude 55° 33′ N, longitude 13° 43′ E and elevation 40-45 m above sea level. The vegetation period, defined as degree days above 5 °C, averaged 209 days during 2007-2016 ([43]). Mean temperature and precipitation were -1.1 °C and 58.5 mm in January, respectively, and 15.9 °C and 67.4 mm in July during the current climate normal period (1961-1990 climate norms - [44]). The soil is a clayey moraine, i.e., an unsorted sediment of glacial origin which is typical for this part of Sweden (Tab. 1). The site is a former arable land distinguished by a humus rich topsoil and a compacted plough pan below at around 30 cm depth. The topsoil layer is characterized as a special form of mull which contains more mineral than organic material ([47]). Larger stones have been removed during an extended period of arable use and repeated ploughing and harrowing have created a homogeneous distribution of the remaining stones in the topsoil.
Tab. 1 - Arable top soil (0-30 cm) origin and textural class (particle sizes < 2 mm), cation exchange capacity (CEC) and degree of base saturation (BS) at pH 7 for soils in the region of the experimental site. The Swedish nomenclature is based on clay content and soil particle size ([1]). The approximate conversion to the international classification system is shown ([9], [11]). Data on CEC and BS for the region is from Eriksson et al. ([10]).
Property | Nomenclature/Units | Value/Range |
---|---|---|
Sediment | - | Unsorted |
Origin | - | Glacial |
Textural class | Swedish nomenclature | Light clay |
International nomenclature | Loam | |
CECpH 7 | cmolc kg-1 dW-1 | 11.0-14.9 |
BSpH 7 | % | 73-86 |
Experimental design
A clonal mix of hybrid aspen was planted on 3.5 ha in spring 1998. One-year-old hybrid aspen plants were planted with a spacing of 3 × 3 m, giving a density of 1100 plants ha-1. In total, 14 plots (30 × 30 m) were planted with eight selected clones delivered from Ekebo Research Station, Forestry Research Institute of Sweden. The space between the plots were 6 m at the minimum and planted with other commercial clones. The plantation was harvested in the winter 2008/2009 when total tree age was 12 years. Four blocks, each including three plots with different management regimes, were identified based on recorded standing biomass after 2 years. The randomized placing of management regimes within blocks was also applied at the end of the second season after clear-cutting. The two plots not used in the study were kept as reserves. Three different management regimes were tested: (i) 4YR, rotation periods of four years with removal of biomass at each harvest; (ii) 8YR, rotation periods of eight years including cleaning in corridors, where biomass was removed, after four years and total harvest of biomass after eight years; (iii) 8+YR, rotation periods of 16 years, where the first eight years were included in the present study, including corridor cleaning with removal of biomass after two years and thinning of the remaining stems to 1100-1200 stems ha-1 after four years. No biomass was taken out at the latter thinning.
The cleaned corridors were 3-3.5 m wide and the strips with shoots were 1-2 m wide. For more details on the different management regimes see Rytter & Rytter ([39]).
Harvested biomass and nutrient removal
Measurement of standing biomass started in autumn 2009 and occurred repeatedly with 1-2 years interval during the forthcoming 8 years. A detailed description of the measurement procedures is given in Rytter & Rytter ([39]). All shoots/trees in sample plots (3-6 plots of 10-15 m2) and net plots, i.e., 30 × 30 m (treatment 8+YR) were measured for breast height diameter over bark (dbh), while height was measured on every fifth shoot/tree. Height of the remaining shoots/trees was calculated from functions produced from the height-measured individuals using the formula (eqn. 1):
where H is stem height, D diameter at breast height (1.3 m), and a and b are constants.
Samples for estimation of stem dry weight were collected simultaneously as diameter measurement. A total of 20-30 shoots/trees of various sizes were collected from each treatment on each sampling occasion. The samples were treated as whole shoots or as a series of discs for determination of wood density and weight. Basic density of wood was recorded on the discs to allow calculation of stem weight for the larger trees ([39]). The water displacement method was used ([31]). Branches were treated separately. After biomass determination of the shoots/trees, relations between diameter and height to dry biomass were constructed using the formulas (eqn. 2, eqn. 3):
where Ws and Wt are stem and branch dry weight, respectively, D is the stem diameter, H is the stem height and c, d, e and f are constants. The result of the biomass measurements (Tab. 2) was also previously presented in Rytter & Rytter ([39]).
Tab. 2 - Harvested and standing biomass during the 8-year study period for the three management regimes. Mean values (n=4) of respective management and measurement occasion are shown. The thinning biomass in the 8+YR regime year 4 was not removed from the plots. Data taken from Rytter & Rytter ([39]).
Management regime |
Type | Year | Age (yrs) |
Biomass (Mg DM ha-1) |
---|---|---|---|---|
4YR | Harvest | 2012 | 4 | 45.82 |
Harvest | 2016 | 4 | 52.48 | |
Total outtake | - | - | 98.31 | |
8YR | Harvest | 2012 | 4 | 25.53 |
Harvest | 2016 | 8 | 67.05 | |
Total outtake | - | - | 92.59 | |
8+YR | Harvest | 2010 | 2 | 17.78 |
Thinning | 2012 | 4 | 15.79 | |
Remaining | 2016 | 8 | 53.62 | |
Outtake, thinning and remaining | - | - | 87.17 |
To produce a representative sample for nutrient analyses of the shoots/trees, sawdust was collected from sawing down to the pith at each 2 m section of the stem, i.e., the sampled discs for biomass determination were used in this purpose for the bigger trees. An equal number of saw tracks was used at each stem section for the individual tree. In this way a volume-based representative sample for the shoot/ tree was achieved.
Carbon (C) as well as macronutrients were analysed on the collected sawdust from respective sample shoot/tree. Total C and nitrogen (N) were analysed by dry combustion of the sample with an elemental analyser (LECO TruMac®, Leco Corp., St Joseph, MI, USA). Samples for analysis of phosphorus (P), sulphur (S), potassium (K), magnesium (Mg) and calcium (Ca) were digested with 65% HNO3 and analysed with ICP-OES (Inductively Coupled Plasma with Optical Emission Spectrometry, Avio 200®, Perkin Elmer, USA).
Soil sampling and analyses
An initial sampling of the mineral soil down to the plough pan, i.e., at approximately 30 cm depth, was conducted in connection with harvest of the original stand and reflected the soil conditions before clear-cutting and application of the different management regimes (4YR, 8YR, 8+YR). A second soil sampling was performed in the winter after eight years with different management. A core sampler with the length 30 cm, outer diameter 25 mm and inner diameter 20 mm was used. Twenty core samples were collected to 30 cm depth on each plot, i.e., on twelve plots on both sampling occasions. Each core sample was divided into two fractions, 0-15 cm and 15-30 cm, and pooled into one sample per depth fraction and plot giving a total of 48 soil samples (2 occasions × 12 plots × 2 depths) which were further processed and analysed for nutrient content and pH. In addition, three soil cores from each plot were taken at the latter sampling occasion for determination of bulk density of the fine earth fraction (particle size <2 mm), gravel volume and coarse organic material in the soil.
Chemical analyses were performed on the homogenized and dried fine earth fraction. Total C (Tot C) and total N (Tot N) were analysed by dry combustion of the sample with an elemental analyser (LECO TruMac). Plant available P and base cations (K, Mg, Ca) were extracted with Mehlich III solution ([2]) and analysed with plasma analysis (ICP-OES Avio 200). Soil samples for analyses of nitrogen in nitrate (NO3-N) and in ammonium (NH4-N) were extracted in 2 M KCl and analysed by spectrophotometry (AutoAnalyzer, AA3®, Seal Analytical GmbH, Norderstedt, Germany). The pH was measured in air-dried soil suspended in deionised water according to the standard SS-ISO 10 390.
The content of inorganic C (carbonates) in Swedish arable soils were considered negligible at pH<6 in a comprehensive study ([10]). The soil pH in the present study ranged between 5.1-5.8 and we assumed that the Tot C values were approximate estimates of soil organic carbon (SOC).
Bulk density of the fine earth to 30 cm soil depth was estimated by using the volume of the core sampler (94.25 cm2). The soil from the sampler was sieved (2 mm hole diameter) to separate fine earth from gravel (2-20 mm) and coarse organic material (2-20 mm). All fractions were dried at 70 °C to constant weight and weighed. The bulk density of the fine earth was calculated by using the formula (eqn. 4):
where ρ is the bulk density of fine earth < 2 mm (g cm-3), M is the mass of fine earth taken with core sampler (g), V is the volume of the undisturbed fine earth (cm3), Mcs is the mass of soil sample, including gravel, taken with core sampler (g), Vcs is the volume of the core sampler (cm3), M2-20mm is the mass of gravel (2-20 mm, g), V2-20mm is the volume of gravel (cm3), ρgr is the mean density of granite (2.650 g cm-3). Eventual soil shrinkage was not compensated for.
Soil nutrient content (Ntcontent) was calculated by multiplying nutrient concentration by the volume of fine earth according to McNabb et al. ([28] - eqn. 5):
where ρ is the bulk density of fine earth < 2 mm (g cm-3), σ2-20mm is the relative volume of gravel, d is the sampling depth (cm), Ntconcentration is the concentration of nutrient (g g-1). The relative stone volume (particle size ≥ 20 mm) to 30 cm depth, was not investigated in the present study. However, an earlier investigation of stoniness (20-200 mm) showed that the relative stone volume was about 4% in arable soils of this region ([41]). Accordingly, it was assumed that the influence of stoniness would only give a minor effect on estimates of nutrient stocks in the present study and that the comparison of management regimes would not be hampered since the soil was of the same origin for all treatment plots.
Statistics
Statistical analyses were performed with R version 3.4.2 ([32]). Analyses of variance (ANOVA) were conducted by using the R built-in function “aov” for balanced data. A two-factor factorial model was used to test for equality in means of bulk density, gravel and organic material in the soil between different management regimes and is described by (eqn. 6):
where yijk is the ijkth observation, μ is the overall mean, si is the fixed effect of the ith treatment, tj is the random effect of the jth block, and εijk is the random error term for observation ijk. The fixed effect of management regime was tested with mean-square of block and treatment as the error term. The same design was used to test for equality in means of nutrient pools (Mg ha-1) and nutrient concentrations (mg kg-1) between management regimes.
A four-factor factorial design was performed to test for equality in means of pH and nutrient concentrations (mg kg-1) between years, depth and the different management regimes (eqn. 7):
where yghijk is the ghijkth observation, μ is the overall mean, qg is the fixed effect of the gth depth, rh is the fixed effect of the hth year, si is the fixed effect of the ith treatment, tj is the random effect of the jth block, and εghijk is the random error term for observation ghijk. The fixed effect of management regime was tested by using mean-square of block and treatment as error term.
Pairwise comparisons were performed by Tukey’s Studentized range test if significant differences were revealed by the main tests. A significant difference was accepted for all tests if the probability for the tested null hypothesis (no difference) was equal to or less than 5 % (p ≤ 0.05).
Results
Nutrient removal with harvests
The biomass was removed from the site at all management operations, except at the thinning year 4 in the 8+YR management regime. The regime with highest biomass removal also showed the highest removal of carbon and nutrients (Tab. 3). For example, 322 kg N ha-1 was removed at the 4YR regime, 223 kg N ha-1 in the 8YR, and only 144 kg N ha-1 in the 8+YR after 8 years. The low outtake of biomass and nutrients in the 8+YR was a result of leaving the material on the site at the second thinning and that no final harvest is to be done before the age of 16 years.
Tab. 3 - Data on the biomass removed at thinning and harvest, including removed amounts of macro nutrients (n=4). Da is the arithmetic breast height diameter (1.3 m) of harvested shoots/trees.
Management regime |
Type | Da (cm) |
Year | Age (yrs) |
Removed biomass, C (Mg ha-1) and nutrients (kg ha-1) | |||||||
---|---|---|---|---|---|---|---|---|---|---|---|---|
Biomass | C | N | P | K | Ca | Mg | S | |||||
4YR | Harvest | 2.90 | 2012 | 4 | 45.82 | 20.91 | 159 | 24.7 | 109 | 190 | 21.0 | 11.4 |
Harvest | 3.26 | 2016 | 4 | 52.48 | 23.95 | 163 | 31.9 | 122 | 292 | 22.7 | 15.1 | |
Total removal | - | - | - | 98.31 | 44.86 | 322 | 56.6 | 232 | 482 | 43.7 | 26.5 | |
8YR | Thinning | 2.61 | 2012 | 4 | 25.53 | 11.65 | 88.3 | 15.1 | 66.9 | 118 | 12.8 | 6.9 |
Harvest | 5.25 | 2016 | 8 | 67.05 | 31.11 | 135 | 26.1 | 111 | 206 | 21.6 | 11.0 | |
Total removal | - | - | - | 92.59 | 42.76 | 223 | 41.2 | 178 | 324 | 34.4 | 17.9 | |
8+YR | Thinning | 1.67 | 2010 | 2 | 17.78 | 8.11 | 144 | 15.6 | 65.8 | 104 | 12.6 | 8.7 |
Thinning | - | 2012 | 4 | 0 | 0 | 0 | 0 | 0 | 0 | 0 | 0 | |
Total removal | - | - | - | 17.78 | 8.11 | 144 | 15.6 | 65.8 | 104 | 12.6 | 8.7 |
Although the 8+YR showed the smallest total outtake of nutrients, the nutrient amount per harvested unit of biomass was highest, 8.1 kg N Mg-1 of biomass. A clear negative relation was seen between average tree diameter, and thereby also shoot age, and nutrient concentration (Fig. 1).
Fig. 1 - The relation between average tree diameter at the harvest or thinning occasion and nutrient concentration in harvested biomass, exemplified by N, P and K. Every data point is a mean from four sample trees of similar size for which the elements were analysed.
Soil nutrient concentrations and pH
A statistically significant but small difference in SOC concentration was detected for different managed root sucker plots with hybrid aspen (Fig. 2a, Tab. 4). The 8YR management regime showed lower SOC concentrations when both depth levels were included in the analyses, but not for each depth alone. The upper 0-15 cm soil contained higher mean SOC concentrations compared to the 15-30 cm level irrespective of management regime. Mean SOC concentrations were 21.2-24.5 and 10.5-15.3 g kg-1 in the upper and lower soil level, respectively.
Fig. 2 - (a) Soil organic carbon (SOC) concentrations (mg kg-1), (b) total nitrogen (Tot N) concentrations (mg kg-1), and (c) CN-ratios in 0-15 and 15-30 cm mineral soil. Means and standard error bars are shown (n=4, each n consisted of 20 subsamples).
Tab. 4 - Results from analyses of variance (ANOVA) of soil pH and nutrient concentrations including two depth levels, three management regimes and two sampling occasions, i.e., at the application of management regimes and eight years later. ANOVA was also performed within each depth level. A difference was accepted as significant if p ≤ 0.05.
Variable | Depth | Management | Year | Change | |
---|---|---|---|---|---|
cm | p-value | p-value | p-value | ||
Tot C | Both | <0.0001 | 0.0476 | - | - |
0-15 | - | 0.474 | - | - | |
15-30 | - | 0.180 | - | - | |
Tot N | Both | 0.0001 | 0.181 | - | - |
0-15 | - | 0.574 | - | - | |
15-30 | - | 0.489 | - | - | |
CN | Both | 0.0085 | 0.108 | - | - |
0-15 | - | 0.654 | - | - | |
15-30 | - | 0.077 | - | - | |
pH | Both | 0.680 | 0.123 | 0.698 | - |
0-15 | - | 0.295 | 0.289 | - | |
15-30 | - | 0.452 | 0.583 | - | |
NH4-N | Both | <0.0001 | 0.670 | <0.0001 | - |
0-15 | - | 0.446 | <0.0001 | increase | |
15-30 | - | 0.144 | 0.326 | - | |
NO3-N | Both | 0.419 | 0.0288 | 0.0010 | - |
0-15 | - | 0.133 | 0.0842 | - | |
15-30 | - | 0.309 | 0.0116 | decrease | |
P | Both | 0.0081 | 0.0598 | 0.656 | - |
0-15 | - | 0.137 | 0.702 | - | |
15-30 | - | 0.560 | 0.849 | - | |
K | Both | <0.0001 | 0.645 | <0.0001 | - |
0-15 | - | 0.496 | <0.0001 | increase | |
15-30 | - | 0.238 | 0.200 | - | |
Ca | Both | 0.775 | 0.374 | 0.426 | - |
0-15 | - | 0.743 | 0.295 | - | |
15-30 | - | 0.550 | 0.995 | - | |
Mg | Both | 0.0189 | 0.552 | <0.0001 | - |
0-15 | - | 0.859 | 0.0005 | increase | |
15-30 | - | 0.655 | 0.0013 | increase |
Concentrations of Tot N in the mineral soil did not differ between management regimes (Fig. 2b, Tab. 4). Differences between depth levels were observed and higher concentrations were found in the top 15 cm soil. Mean Tot N concentrations were 1.6-1.9 g kg-1 in the top 15 cm soil and 0.9-1.4 g kg-1 in the lower soil depth.
The soil CN ratio was not affected by management regime (Fig. 2c, Tab. 4). Mean CN ratios were higher in the 0-15 cm soil level and ranged between 12.3-12.9, compared to 11.2-12.0 in the 15-30 cm level.
Soil pH did not change significantly over time and did not differ between management regimes or depth levels. Mean pH values were 5.4-5.6 at the time of clear cutting and 5.5-5.8 after 8 years of management (Fig. 3a, Tab. 4).
Fig. 3 - (a) pH and (b) concentrations (mg kg-1) of nitrogen in ammonium (NH4-N) and nitrate (NO3-N) in 0-15 and 15-30 cm mineral soil. The x-axis shows concentrations before application of management regimes and the y-axis shows concentrations eight years later. Means and standard error bars are shown (n=4, each n consisted of 20 subsamples). The dotted line indicates no concentration difference over time.
The vertical distributions of ammonium and nitrate concentrations in the mineral soil changed differently during the study period (Fig. 3b). An increase in NH4-N concentrations could be seen in the top 15 cm soil in all management regimes, but no differences between the regimes were observed (Tab. 4). Mean concentrations ranged between 9.9 and 13.0 mg kg-1 after eight years. The NH4-N concentrations in the deeper 15-30 cm soil were lower, 2.9-4.2 mg kg-1, and were unaffected by management regime and time. Nitrate concentrations decreased in both soil depths, but statistically significant only in the lower 15-30 cm soil (Fig. 3b, Tab. 4). A small but statistically significant difference between the 4YR and 8YR regime was found.
Soil phosphorus concentrations were relatively stable during the study period and no management effects were detected (Fig. 4, Tab. 4). Somewhat higher P concentrations were found in the top 0-15 cm soil compared to the lower soil layer. The concentrations during the study period ranged between 150-180 and 120-150 mg kg-1 in the 0-15 and 15-30 cm soil depth, respectively.
Fig. 4 - Concentrations (mg kg-1) of plant available phosphorus (P) and base cations (K, Ca, Mg) in 0-15 and 15-30 cm mineral soil. The x-axis shows concentrations before application of management regimes and the y-axis shows concentrations eight years later. Means and standard error bars are shown (n=4, each n consisting of 20 subsamples). The dotted line indicates no concentration difference over time.
Base cation (K, Ca, Mg) concentrations in the mineral soil were not affected by management regime (Fig. 4, Tab. 4). Concentrations of K and Mg increased during the study period while Ca concentrations were stable in all regimes. Potassium concentrations increased in the top 0-15 cm soil and Mg concentration increased in both soil depths. Concentrations of K and Mg varied with soil depth, while concentrations of Ca were similar. Mean K concentrations were 190-210 and 55-67 mg kg-1 in the 0-15 and 15-30 cm depth, respectively, and corresponding mean Mg concentrations were 160-190 and 120-160 mg kg-1 in all management regimes. Mean Ca concentrations ranged between 900-1800 mg kg-1, including all management regimes, depths and years.
Bulk density and soil nutrient pools
Bulk density of the fine earth and relative gravel volume of the soil to 30 cm depth were estimated at the end of the current study period. No significant differences in bulk density caused by different management regimes could be detected (Tab. 5). The relative gravel volume was low in all regimes as well as the content of coarser organic material, i.e., small roots and not fully decomposed litter.
Tab. 5 - Bulk density of fine earth (particle size <2 mm), relative volume of gravel (2-20 mm) and content per soil volume of coarse organic material (2-20 mm) to 30 cm soil depth after eight years different management of second generation hybrid aspen. Mean values (n=4 where each n consisted of three subsamples), standard errors (Std.err.) and p-values for treatment means are shown.
Management regime |
Fine earth (g cm-3) |
Gravel relative volume (%) |
Organic (g cm-3) |
|||
---|---|---|---|---|---|---|
Mean | Std.err. | Mean | Std.err. | Mean | Std.err. | |
4YR | 1.12 | 0.03 | 2.65 | 0.63 | 0.0032 | 0.0014 |
8YR | 1.18 | 0.05 | 1.98 | 0.40 | 0.0066 | 0.0025 |
8+YR | 1.20 | 0.06 | 1.36 | 0.24 | 0.0032 | 0.0006 |
p-value | 0.491 | - | 0.162 | - | 0.200 | - |
The calculated soil nutrient pools did not differ between the various management regimes for root suckers of hybrid aspen (Tab. 6). The slightly lower SOC concentration observed in the 8YR regime (Fig. 2a) was reflected in the SOC pool but it was not statistically significant. The CN ratios calculated from SOC and Tot N pools (Mg ha-1) included the soil depth 0-30 cm and showed similar figures, i.e., 12.2-12.3, in all regimes. The pools of P, K and Mg were of the same magnitude, 0.4-0.6 Mg ha-1, in all management regimes, while the Ca pools were about ten times larger.
Tab. 6 - Soil pools (Mg ha-1 to 30 cm depth) of SOC and Tot N, CN-ratio, NH4-N, NO3-N, P and base cations (K, Ca, Mg) after eight years different management of second generation hybrid aspen. Mean values (n=4, each n consisting of 20 subsamples), standard errors (Std.err.) and p-values for treatment means are shown.
Element (Mg ha-1) |
Stats | Management regime | p-value | ||
---|---|---|---|---|---|
4YR | 8YR | 8+YR | |||
SOC | Mean | 64.70 | 54.80 | 65.90 | 0.095 |
Std.err. | 6.30 | 5.42 | 4.62 | - | |
Tot N | Mean | 5.35 | 4.47 | 5.44 | 0.375 |
Std.err. | 0.63 | 0.40 | 0.49 | - | |
CN | Mean | 12.20 | 12.30 | 12.20 | 0.404 |
Std.err. | 0.52 | 0.49 | 0.29 | - | |
NH4-N | Mean | 0.0217 | 0.0272 | 0.0305 | 0.997 |
Std.err. | 0.0013 | 0.0013 | 0.0023 | - | |
NO3-N | Mean | 0.0119 | 0.0057 | 0.0082 | 0.342 |
Std.err. | 0.0019 | 0.001 | 0.0019 | - | |
P | Mean | 0.500 | 0.570 | 0.477 | 0.673 |
Std.err. | 0.018 | 0.064 | 0.096 | - | |
K | Mean | 0.417 | 0.437 | 0.489 | 0.683 |
Std.err. | 0.05 | 0.043 | 0.058 | - | |
Ca | Mean | 5.64 | 3.45 | 4.83 | 0.919 |
Std.err. | 1.24 | 1.11 | 0.78 | - | |
Mg | Mean | 0.517 | 0.497 | 0.615 | 0.799 |
Std.err. | 0.057 | 0.108 | 0.091 | - |
Discussion
We found few significant effects on mineral soil properties related to the different management regimes after eight years in root sucker stands with hybrid aspen (hypothesis 1). Different harvest intervals and different degrees of thinning with removal of biomass (Tab. 3) caused no differences in SOC pool sizes (Tab. 6) and SOC concentrations showed only a minor statistical significant divergence for the 8YR from the other regimes (Fig. 2a, Tab. 4). This minor difference might be due to the removal of many shoots and a large part of the biomass in corridor cleaning after 4 years in the 8YR treatment, thereby leaving gaps in the corridors for some time, which probably resulted in less production of leaf and root litter. However, the SOC pool was not affected so this tendency needs to be followed to get accurate information on SOC development.
Few studies have been conducted on the impact of harvesting or thinning intervals on soil properties in poplar plantations, and none in hybrid aspen stands so far. It has been suggested that short rotation forestry in general would have a positive impact on C sequestration ([14]) and that hybrid poplar plantations with rotations longer than four years could act as C sinks ([5]). Other results from stem harvesting or thinning impact on mineral soil C stocks in temperate forests are variable and more studies are requested on the subject ([29]). The meta-analysis by Nave et al. ([29]) found no significant overall change in C storage in mineral soils caused by stem harvesting. Likewise, thinning studies with various intensities and time intervals revealed either no effects on or minor decreases of C pools in the mineral soil ([34], [30], [21]), but the literature on harvesting and thinning effects on soil C in temperate forests refers to a high degree to conifers. Comparative studies on this subject revealed that hardwoods in general lose more forest floor C than coniferous/mixed stands, while the situation in the mineral soil seems to be more dependent on soil type ([29], [21]). The different management regimes for root sucker generated by hybrid aspen tested in the present study did not affect the SOC pool sizes in the mineral soil after eight years. However, it is important to follow effects of management on soil properties over several rotations.
Removed biomass quantities were largest in the management regimes with the shortest rotations, 4YR and 8YR, and it reflected the extent of C and nutrient removal (Tab. 3). The dimensions of harvested stems influenced the amounts removed nutrients (Fig. 1). Removed quantities of N were largest for the lower diameter shoots and would accordingly affect the 4YR regime most (Fig. 1, Tab. 3). However, Tot N soil concentrations (Fig. 2b, Tab. 4) and pools (Tab. 6) of the 4YR regime did not differ from those of the other regimes after two complete rotations (hypothesis 2). There was no productivity decline observed in the two consecutive 4-year rotations, which suggests that there was no shortage of N ([39]). The CN ratios were similar, i.e., around 12 based on amounts (Mg ha-1), in all management regimes (Tab. 6). These CN ratios were of the same magnitude as those measured in a comprehensive study of Swedish arable soils, containing 2034 sampling points across Sweden with a mean CN ratio of 11 ([10]). Thus, the soil fertility in our studied plantation was in the same range as fertile arable soils almost 20 years after afforestation with hybrid aspen. Increasing ratios will be expected upon afforestation due to the input of forest litter with higher CN ratios compared to annual crops, uptake of inorganic N and cessation of N-fertilization ([20], [35]), but the effect of annual crop cultivation could remain for 30 years or even longer ([22], [33]).
Decreases in pH may be expected upon afforestation ([20], [33], [25], [42]). Swedish arable soils had a mean pH of 6.3 ([10]) which is somewhat higher than the pH measured in our plantation, i.e., 5.5-5.8 at the latter sampling. However, we found no significant time effect on soil pH during our study interval and no differences caused by management regime (Fig. 3a, Tab. 4).
Ammonium is released by the mineralization process and is an important form of N utilized by plants. The significant increase in the top 15 cm mineral soil in all management regimes can thus be explained by enhanced uptake of NH4-N by fast-growing hybrid aspen from deeper horizons and recirculation by decomposing litter at the surface (Fig. 3b, Tab. 4). It is by now well-known that tree species influence the size of the nutrient pools and the distribution among soil horizons ([6]). It can also be concluded from the increased or unchanged concentrations in both investigated soil depths in the present study that the rate of N uptake did not exceed the rate of mineralization in these layers. The effect on NO3-N concentrations was the opposite, i.e., a declining trend in all regimes (Fig. 3b, Tab. 4). This could be an indication of leakage of the ion. However, an analysis of vertical distribution of nutrients in soils found an extremely variable distribution for NO3-N which makes interpretations difficult ([17]).
Afforestation of cropland may lead to decreases in P concentrations and P pools in the upper mineral soil according to a meta-analysis ([8]). However, we found that the plant available P concentrations were in the range of 120-180 mg kg-1, including both soil depths and all management regimes (Fig. 4, Tab. 4), which was higher than the mean concentrations of 80 mg kg-1 estimated for Swedish arable soils (ammonium lactate-acetate extracted - [10]). Thus, there were apparently no depletion of plant available P and the concentrations remained stable during the studied period.
Base cation concentrations and pools in the mineral soil did not differ between management regimes, but general decreases in concentrations in the deeper soil layer and increases in the shallower layer were observed for K and Mg, but not for Ca (Fig. 4, Tab. 4, Tab. 5). This implies a plant mediated redistribution of K and Mg by plant uptake in deeper soil and release through litter decomposition at the surface which has also been suggested in earlier studies ([3], [17], [42]). Calcium is important for regulation and development of plant growth as well as in soil buffering systems. It was the most abundant cation found in the soil in the present study (Tab. 6), but it showed also the largest removal figures (Tab. 3). However, Ca is required in small amounts in plants ([15]) and the concentrations measured were stable over time (Fig. 4).
An estimate of bulk density is important for assessing the size of nutrient pools in soils. Soil bulk density could be affected by compaction, activity of soil organisms, presence of roots and soil organic material ([7]). Differences in bulk density between management regimes in the present study may be expected due to various intensity in harvests and thinning measures and different stand densities. This will cause differences in the supply of above ground litter, the density of roots and root litter supply, soil temperature and humidity which together probably will cause differences in the amounts of SOC. However, no statistically significant differences in bulk density between the regimes were observed so far (Tab. 5).
Conclusions
The different management regimes in root-sucker generated hybrid aspen resulted in few significant effects on mineral soil characteristics (hypothesis 1), despite large variations in removed quantities of biomass and nutrients (hypothesis 2). The only exception was a slightly lower SOC concentration in the 8YR regime. Thus, it can be concluded that the management measures with various harvesting and thinning intensities did not influence soil characteristics differently during the first eight years after initiation. It is however important to follow the development of soil characteristics in these plantations during several rotations since short rotation forestry with hybrid aspen is a comparatively new silvicultural approach and the long-term impact on soil properties is unclear.
Common for all management regimes were the unchanged SOC and nutrient pools in the upper 30 cm mineral soil. pH and bulk density were also unaffected during the studied period. Afforestation effects on the vertical distribution of nutrients, i.e., redistribution of NH4-N, K and Mg from deeper to shallower soil by plant uptake and release through litter decomposition, were observed in all management regimes. A different effect was noted for NO3-N where a declining trend was observed in both the investigated soil depths. This could indicate a leakage of the ion, but NO3-N distributions in soils are variable which makes interpretations difficult.
Acknowledgements
The authors wish to thank Frosten Nilsson and Fredrika Rytter for their help with the soil sampling, as well as the personnel at the Ekebo research station of the Forestry Institute of Sweden for processing the wood samples. We are also grateful for valuable comments on the manuscript from three anonymous reviewers. The study was financially jointly supported by the Swedish Energy Agency, the frame program of the Forestry Research Institute of Sweden and Rytter Science.
References
Gscholar
Gscholar
Gscholar
Gscholar
Gscholar
Gscholar
CrossRef | Gscholar
Gscholar
Gscholar
Gscholar
Authors’ Info
Authors’ Affiliation
Rytter Science, Backavägen 16, SE-268 68, Röstånga (Sweden)
The Forestry Research Institute of Sweden (Skogforsk), Ekebo 2250, SE-268 90, Svalöv (Sweden)
Corresponding author
Paper Info
Citation
Rytter R-M, Rytter L (2018). Effects on soil characteristics by different management regimes with root sucker generated hybrid aspen (Populus tremula L. × P. tremuloides Michx.) on abandoned agricultural land. iForest 11: 619-627. - doi: 10.3832/ifor2853-011
Academic Editor
Werther Guidi Nissim
Paper history
Received: May 17, 2018
Accepted: Aug 11, 2018
First online: Oct 04, 2018
Publication Date: Oct 31, 2018
Publication Time: 1.80 months
Copyright Information
© SISEF - The Italian Society of Silviculture and Forest Ecology 2018
Open Access
This article is distributed under the terms of the Creative Commons Attribution-Non Commercial 4.0 International (https://creativecommons.org/licenses/by-nc/4.0/), which permits unrestricted use, distribution, and reproduction in any medium, provided you give appropriate credit to the original author(s) and the source, provide a link to the Creative Commons license, and indicate if changes were made.
Web Metrics
Breakdown by View Type
Article Usage
Total Article Views: 18262
(from publication date up to now)
Breakdown by View Type
HTML Page Views: 14733
Abstract Page Views: 1153
PDF Downloads: 1882
Citation/Reference Downloads: 8
XML Downloads: 486
Web Metrics
Days since publication: 2123
Overall contacts: 18262
Avg. contacts per week: 60.21
Article Citations
Article citations are based on data periodically collected from the Clarivate Web of Science web site
(last update: Nov 2020)
Total number of cites (since 2018): 3
Average cites per year: 1.00
Publication Metrics
by Dimensions ©
Articles citing this article
List of the papers citing this article based on CrossRef Cited-by.
Related Contents
iForest Similar Articles
Research Articles
Soil stoichiometry modulates effects of shrub encroachment on soil carbon concentration and stock in a subalpine grassland
vol. 13, pp. 65-72 (online: 07 February 2020)
Research Articles
Carbon storage and soil property changes following afforestation in mountain ecosystems of the Western Rhodopes, Bulgaria
vol. 9, pp. 626-634 (online: 06 May 2016)
Research Articles
Impact of deforestation on the soil physical and chemical attributes, and humic fraction of organic matter in dry environments in Brazil
vol. 15, pp. 465-475 (online: 18 November 2022)
Short Communications
Variation in soil carbon stock and nutrient content in sand dunes after afforestation by Prosopis juliflora in the Khuzestan province (Iran)
vol. 10, pp. 585-589 (online: 08 May 2017)
Research Articles
Comparison of soil CO2 emissions between short-rotation coppice poplar stands and arable lands
vol. 11, pp. 199-205 (online: 01 March 2018)
Research Articles
Effects of tree species, stand age and land-use change on soil carbon and nitrogen stock rates in northwestern Turkey
vol. 9, pp. 165-170 (online: 18 June 2015)
Research Articles
Estimating changes in soil organic carbon storage due to land use changes using a modified calculation method
vol. 8, pp. 45-52 (online: 17 June 2014)
Research Articles
Potential relationships of selected abiotic variables, chemical elements and stand characteristics with soil organic carbon in spruce and beech stands
vol. 14, pp. 320-328 (online: 09 July 2021)
Research Articles
Dynamics of soil organic carbon (SOC) content in stands of Norway spruce (Picea abies) in central Europe
vol. 11, pp. 734-742 (online: 06 November 2018)
Research Articles
The manipulation of aboveground litter input affects soil CO2 efflux in a subtropical liquidambar forest in China
vol. 12, pp. 181-186 (online: 10 April 2019)
iForest Database Search
Search By Author
Search By Keyword
Google Scholar Search
Citing Articles
Search By Author
Search By Keywords
PubMed Search
Search By Author
Search By Keyword