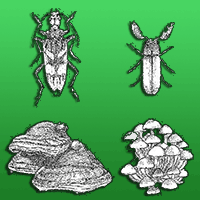
Linking deadwood traits with saproxylic invertebrates and fungi in European forests - a review
iForest - Biogeosciences and Forestry, Volume 11, Issue 3, Pages 423-436 (2018)
doi: https://doi.org/10.3832/ifor2670-011
Published: Jun 18, 2018 - Copyright © 2018 SISEF
Review Papers
Abstract
Deadwood is a substantial component of forests playing a central role in many ecosystem processes. It provides habitats for a multitude of wood-dependent organisms, maintaining the ecosystem health and reducing the effect of natural disturbances. Deadwood is recognized as an indicator of local species diversity and contributes to the global carbon pools and nutrient cycles. Despite its importance, how saproxylic communities respond to deadwood dynamics across multiple spatial and temporal scales is still not clear. With the present review, we aim to summarize the effects of deadwood characteristics on the diversity and composition of saproxylic insects and fungi, with focus on European forests. We also discuss the influence of other biotic and abiotic components that indirectly affect these communities by altering wood continuity and variety. Niche differentiation is the main ecological driver of saproxylic organisms, as the presence of multiple microhabitats supports differently specialized taxa. The assemblage and richness of these saproxylic communities within forest ecosystems can be considered as indicators of climate-smart forestry trajectories. This aspect deserves to be regarded as a major target in sustainable forest management plans, especially in mountain areas, where the conservation of threatened species and the promotion of biodiverse forests are considered a priority.
Keywords
Deadwood Accumulation, Deadwood Decomposition, Saproxylic Insects, Saproxylic Fungi, European Forests, Conservation-oriented Forestry
Introduction
Deadwood is considered a key element of forest ecosystem function and stand structure. It plays a relevant role for the maintenance of biodiversity and complex trophic chains, influencing the natural regeneration of trees and the soil development ([34]). Deadwood is also involved in a variety of ecosystem processes, like the overall carbon sequestration ([77]), which is a primary foundation for climate change mitigation and adaptation, and for approaching climate-smart forestry ([93]). Indeed, most of the plant biomass in forests feeds into detritivorous food chains, supporting saproxylic insects and fungi, thus nutrient cycling and ecosystem stability. The trophic relationships between saproxylic organisms change over time, as deadwood decomposition progresses ([99]).
The role of deadwood in forests has been widely documented, and can be divided into four, inter-related categories: (1) increase the tree productivity; (2) provide habitats and structures to maintain and increase the biological diversity; (3) reduce the hydrological risk in steep slopes, controlling the water run-off and the stream flows; (4) store carbon in a medium-term perspective. The importance of each of these functions varies throughout the bio-geo-climatic regions, since they are influenced by the types of natural disturbance, the intensity and type of silvicultural activities applied, but also in relation to the moisture regime ([83]).
Deadwood is commonly classified according to its thickness in coarse woody debris (CWD), when the mean diameter is higher than 10 cm, in fine woody debris (FWD), with the mean diameter ranging from 5 to 10 cm, and very fine (VFWD), when the mean diameter is smaller than 5 cm ([19], [48], [42]). During the last years, CWD has been extensively studied because of its longer persistence on the forest floor if compared to fine debris, representing a suitable substrate for different environmental functions: it provides seed germination sites ([34]), serves as moisture reservoir during droughts ([91]) and supplies habitats for many birds ([23]) and mammals ([55]). Moreover, saproxylic organisms, including taxa of both fungi and insects, strongly rely on the availability of woody resource to complete their life cycle ([91]).
Deadwood occurrence and continuity is strongly related to local conditions as it depends on natural tree mortality due to senescence, disease and outbreaks, as well as catastrophic disturbance events, such as fires, storms and floods ([34], [62]). In general, deadwood amounts are notably higher in natural and unmanaged forests, where wood harvesting is limited or completely absent. These ecosystems are often located in mountain and remote areas, where human activities have been abandoned for long times due to changes in cultural and economic interests ([16], [63]). Within these ecosystems, the assessment of deadwood production and decomposing dynamics may improve the current knowledge on potential impacts of global change on forest structure and related ecosystem services.
Deadwood monitoring (i.e., fine and coarse woody debris, standing and downed dead trees) is particularly important in semi-arid environment, where tree death by disturbance and depletion by combustion may result from the combined effects of factors and processes induced by climate and land use changes. Forest fires in Mediterranean Europe are more often becoming catastrophic, and risk is increasing for the expansion of forests due to the depopulation of rural areas, the accumulation of fuel loads due to the lack of forest management, and the more severe droughts due to rising temperature, which add to human-induced fires. Fire management (prescribed burning and managed wildfire) and mechanical fuel reduction may reduce the risk of forest fire, though the ecological benefit should consider the roles of deadwood ([10]).
For generations, people have looked at deadwood as something to be removed from forests, either to use it as fuel, or simply as a necessary part of “correct” forest management. Dead trees are supposed to harbor disease and even veteran trees are often regarded as a sign of poor forest management. In international (including Europe) political processes, deadwood is increasingly being accepted as a key indicator of naturalness in forest ecosystems, and woody detritus are indeed a critical component of natural forests ([34]). Many governments have recognized the need to preserve the range of forest values and are committed to those processes that may help reverse the current decline in forest biodiversity. This can be done by including deadwood in national biodiversity and forest strategies, monitoring deadwood, removing perverse subsidies that pay for its undifferentiated removal, introducing supportive legislation and raising awareness. In order to implement forest ecosystem management strategies, quantifying the amount and quality of woody debris and their decomposition patterns becomes an important target to plan future forests ([78]).
Despite the critical role of deadwood in achieving synergies between carbon sequestration and biodiversity conservation, quantitative data need to be integrated on its distribution and turnovers across different biomes, particularly in South-European forest types. Moreover, the use of deadwood as indicator of biodiversity requires a deeper evaluation of the relationships between wood characteristics and saproxylics’ habitat preferences. Deadwood volume per se is not sufficient to infer patterns of saproxylic diversity and further traits should be taken into account for a suitable use of this indicator, both at local and landscape scales ([50]). In Mediterranean forests, recent studies have confirmed the positive effect of the heterogeneity in types and frequencies of microhabitats on biodiversity indicators, but have also highlighted the link between the complexity of forest stands and the abundance and diversity of saproxylic species ([65], [68]).
We aim to provide a comprehensive view on the importance of deadwood in sustaining forest biodiversity and productivity, with a particular emphasis on European forest ecosystems. We highlight the role of woody debris in carbon and nutrient cycling and soil development. Furthermore, we review the colonization and succession patterns of saproxylic insects and fungi, stressing the common aspects and the divergences in their life cycles, also in relation to specific substrate traits. The influence of different biotic and abiotic components in the dynamics of saproxylic communities is also discussed. Finally, based on current knowledge, we elucidate the role and the future challenges of sustainable forest management for the conservation of saproxylic communities in mountain environments, since these habitats are among the most threatened by global change.
Geographical context and article selection
This review was centered on forest ecosystems and saproxylic communities of the temperate and Mediterranean biogeographic zones of Europe. Saproxylic invertebrates and fungi are the most important portion of the wildlife linked to the necromass and their relationships with deadwood components have a strong impact on wood decay processes.
It has been estimated that biodiversity related to deadwood alone represents about 30% of the global biodiversity of a forest environment, even reaching 50% in some groups, such as Coleoptera. In Europe, almost 1.500 species of fungi and 4.000 species of Coleoptera are deadwood-dependent ([91]). Nevertheless, many saproxylic organisms are also threatened ([60]).
In order to scrutinize studies concerning the effects of deadwood characteristics on species diversity and the composition of saproxylic insects and fungi in forest ecosystems, we relayed on titles, abstracts and introductive sections. Specifically, (i) deadwood as an indicator of diversity of species, carbon stock and bio-geochemical cycles; (ii) influence of biotic and abiotic components on saproxylic communities conditioned by continuity and variety of deadwood; (iii) how sustainable forest management may affect the conservation of saproxylic communities in mountain forest environments.
We conducted a search on the Web (Scopus, Google Scholar, ResearchGate) for documents that corresponded to the three review subjects (deadwood, saproxylic insects and saproxylic fungi). For each subject, considering the criteria (i, ii and iii), the following keywords were chosen: deadwood (forests, deadwood, wood decomposition, deadwood decay, mountain forest); saproxylic insects (biodiversity, coleoptera, saproxylic beetles, saproxylic fauna, saproxylic insects); saproxylic fungi (wood-inhabiting fungi, saproxylic fungi, decomposer fungi).
This research was conducted in 2017, considering 100 studies. Documents that did not meet at least one of the chosen criteria were excluded. Forty-five (45) papers (45% of the total) were used for the “saproxylic fungi and invertebrate” section, covering the time span between 2006 and 2017. Earlier studies were taken into account whether they represented reference knowledge on the topic.
Deadwood accumulation and decay
Deadwood comes from large branches, stumps, tree tops, whole dead trees occurring on the forest floor, and standing dead trees. Both extreme events (e.g., strong winds) and more chronic and small-scale disturbances may cause tree damage (broken branches and tops), stem breakage and tree fall. Soil depth and moisture content, geographic location, stand structure, tree age, location in a stand, aspect and tree species affect the amount of deadwood and its spatial distribution. Often wind is the final agent causing tree fall, even if the original damage to trees being induced by other abiotic factors (e.g., fire), as well as biotic factors (e.g., pest and disease). Forest fires produce large amount of deadwood, either directly or indirectly by making trees more susceptible to wind, disease or insect attacks. Yet, insect outbreaks and disease epidemics can directly cause the tree death. Suppression and competition may also increase deadwood occurrence. Suppressed trees that exhibit slow growth are stressed and susceptible to attack by pest and disease. Finally, also senescence may contribute to the susceptibility of trees to insect, disease and wind damages.
Tree species, time since death, age at time of death, cause of death, tree position (standing, downed) and size, as well as the surrounding environmental conditions, may influence the volume and quality of deadwood and its relevance for forest organisms. Small logs and branches do not decay in the same way as large trunks, which may lead to less habitat variety.
The process of deadwood recycling can take hundreds of years to complete and includes three main phases ([89]). In the initial colonisation phase, the wood is invaded by saproxylic organisms (often beetles associated with fungi), which attack the wood when it is still hard. Subsequently, in the decomposition phase, the saproxylic organisms (primary and secondary) slowly feed on material that has already been partially converted by colonisers. In the final and long humification phase, the saproxylic organisms are progressively replaced by scavenging organisms, which incorporate wood residues into the soil.
More in detail, the initial phase is characterized by the mechanical cutting of the woody tissues by insects; the consequent wood fragmentation increases the surface exposed to the action of fungi and abiotic agents. Moreover, the progressive enzymatic digestion involves simple sugars, including monosaccharides and disaccharides, as well as complex components, such as cellulose. Lignin is finally degraded by the action of fungi and, to a lesser extent, bacteria ([3]). Components of each trophic level act on the material already used by the previous trophic level, to be made available to the next levels, so that the decomposition is completed.
Apart from the decomposer communities, decay rates in wood are determined by the interactions between (1) wood quality and (2) abiotic factors ([17]), therefore, markedly vary along the mountain slopes and latitude.
Wood moisture is an important driver of wood decomposition, while decomposing organisms, although they differ in preference to substrate conditions, all share the need for oxygen and water ([17]). Fungi are inactive with less than 20% of humidity, while between 80% and 100% the enzymatic activity due to lack of oxygen is limited ([101]).
Most of the organic matter deriving from tree decay consists of two components: the photosynthetic tissues, such as leaves and needles, which are periodically replaced by plants and deposited on the ground; the dead trunks or parts of them, decomposed by saproxylic organisms. In forest ecosystems, organic matter is destined to enter the processes of humification and mineralization that lead to topsoil formation, maintaining the long-term fertility.
The presence of deadwood alters the chemical characteristics of the soil by increasing calcium, magnesium and total concentrations of nitrogen ([44]), whereas soil pH decreases ([88]). Advanced wood decay phases break down considerable amounts of organic matter and organic carbon dissolved in soil ([102]). However, how deadwood affects abiotic and biotic characteristics in its sphere of influence is not well understood ([30]). Several authors have suggested that soil contact accelerates decomposition of deadwood, but the biotic soil response is unclear ([29]). The persistence in time of decaying wood and its slow decomposition allow the development of long-term interactions between deadwood and soil ([34], [30]).
The amount and the quality of deadwood provide information on the intensity of past anthropic and natural disturbances and the conditions for the accumulation of deadwood across decades to come ([100]). In managed forests, the quantity of deadwood represents between 2% and 30% of the volumes occurring in unmanaged forests ([26]). For this reason, the site-specific conditions influencing the accumulation and degradation of deadwood are considered important structural indicators of naturalness ([40]). Nevertheless, the role of deadwood has not received the proper attention in southern Europe ([53], [30]). In general, past forest management was mainly focused on timber production and deadwood was commonly removed from the forest mainly for local purposes and domestic uses ([30]). It was also removed to limit fuel load (minimizing fires) and facilitate the silvicultural interventions ([15]). Current forest management practices are committed to increase deadwood amount ([33]), considering that the volume of standing and downed deadwood is one of the nine pan-European indicators for sustainable forest management (Criterion 4: Maintenance, Conservation and Proper Valorization of Biological Diversity in Forest Ecosystems). Nevertheless, in Mediterranean and mountain conditions, where forest fires and hydrological risks are common, increasing the amount of deadwood may have ambiguous consequences in terms of environmental benefits, at least in the current complex disturbance scenario.
In conclusion, the presence of large amounts of organic matter in various degradation phases leads to the availability of a large number of ecological niches. Nevertheless, the shortening of harvesting rotation in modern forests has caused a decrease in deadwood amount and variety, as well as age distribution of living trees and decomposition stage of woody debris, which is critical for many saproxylic species ([36]). However, conservation-oriented siliviculture is recently revaluating the possibility to retain suitable woody debris to promote biodiversity in forests.
Mountain forest ecosystems and saproxylic biodiversity
Mountain areas, with rugged terrain and steep slope, represent refugial areas for old-growth forests and wood-dependent species, being zones unsuitable for modern agriculture. Remnant old-growth forests are structurally complex ecosystems with consistent amount of decomposing wood, providing special habitats and trophic niches for many species, such as birds, mammals, invertebrates, lichens, bryophytes, and particularly saproxylic organisms ([67]). Saproxylic is the term used to denote those species that depend on deadwood at least in one stage of their life cycle. They represent a high portion of the biodiversity occurring in deadwood ([91]).
In mountain areas, the seasonal progressions of temperature, precipitation, and solar irradiance strongly determine the availability of resources for vegetation. The climate is characterized by cool, wet winters and frequent dry summers with high solar irradiance, especially in the Mediterranean context. Mediterranean mountain forests are relatively rich in tree species diversity; deciduous stands at the lower altitude are mainly dominated by oak species, while European beech forests occurs from 1.000 m a.s.l. The occurrence of coniferous species is also common, often mixed with broadleaves in the Mediterranean context, while characterizing pure stands in the sub-Alpine ecoregions.
In general, latitude and topographic traits strongly affect the tree composition, raising the boundaries of the various belts, as passing from North to South and from North- to South-facing slopes ([82]).
Although the landscape structure of mountain forests is heterogeneous, that mosaic is often less fragmented by human activity in comparison to lowland forests. In mountain forest ecosystems, deadwood amounts range between 14 and 150 m3 ha-1, in the natural and semi-natural forests, with percentages varying between 5% and 30% of standing biomass ([54]). In some protected areas of Mediterranean mountains, where European beech forests are prevalent, the total amount of deadwood is about 130 m3 ha-1 ([54]).
The anthropic influences on temperate forests have led to profound changes in stand structures over the centuries and the decreased occurrence and diversity of deadwood in mountain forests mirror this legacy. These changes have deeply affected the diversity and structure of mountain forest communities, influencing organisms that depend on deadwood ([91]).
The interaction between deadwood traits and saproxylic organisms
Deadwood characteristics (volume, species, variety, age distribution) are often used as indicators of forest biodiversity as they are strongly related to species-specific traits of saproxylic organisms ([91]).
Communities of saproxylic fungi and beetles associated with deadwood change with time according to the stage of wood decay, which reflects changing physico-chemical wood conditions and the contact with topsoil. Similarly, different tree species host specific saproxylic communities. However, the degree of specialization is species-dependent and often varies according to the decay stage. For example, the first colonizers, bark beetles, are specialized on a single tree species or genus ([22]). As the decomposition process progresses, the specificity of the linkage between saproxylic and tree species decreases. In spite of this, the difference in species composition between coniferous and hardwood trees remains consistent throughout the decomposition process ([91]).
Saproxylic invertebrates
Saproxylic insects are a group of organisms that may experience significant increase in resource availability following pest outbreaks, which induce tree mortality, as well as other natural disturbances that cause canopy damage and woody detritus (e.g., wildfires, windstorms, heavy snowfalls).
The decomposition process progresses from species of invertebrate more or less dependent on deadwood to species of invertebrates totally dependent; consequently, at advanced stages of decay there is no longer a distinct species differentiation ([18]). Many studies have focused on the succession of insects that are dependent on deadwood, namely in boreal regions and on the change in the physical and chemical properties of deadwood. The succession of fauna has been found to be very similar in silver fir and European beech in most of European studies (Tab. 1).
Tab. 1 - The four stages of wood decomposition and associated saproxylic families in European beech and silver fir (modified from [18]).
Succession | Stage | Decay | Saproxylic families |
---|---|---|---|
Succession in European beech |
First stage | Wood decay 1 to 4 years since the death of the tree | Cerambycidae, Buprestidae, Curculionidae |
Second stage | Wood decay 3 to 7 years since the death of the tree | Cerambycidae, Anobiidae, Buprestidae, Curculionidae and predators: Cucujidae, Trogositidae, Cleridae | |
Third stage | 3 to 10 years since the death of the tree | Cerambycidae, Lucanidae, Tenebrionidae, Elateride | |
Fourth stage | Wood decay 10 years since the death of the tree - crumbly wood | Larvae of Diptera and various beetles, Pselaphidae, Ptiilidae and various invertebrates lumbricids and Enchytraeidae | |
Succession in silver fir |
First stage | Immediately after the death of the tree, the wood is little affected and the color has not changed (1 to 5 year) | Cerambycidae, Curculionidae, Lucanidae, Elateridae, predators: Cleridae, Staphylinidae and Diptera |
Second stage | Wood decay 5 to 7 years since the death of the tree | Fungal invasions. Cerambycidae, Curculionidae, Lucanidae, Elateridae, Cleridae predators, Staphylinidae, Carabidae, and Diptera |
|
Third stage | The cortex is largely detached (6 to 10 year) | Lignicolous fungi that form fruiting bodies. Elateridae, Cerambycidae, Staphylinidae, Diptera and ants | |
Fourth stage | Wood in advanced state of decomposition | Lucanidae, Elateridae, Staphylinidae, Diptera. Massive invasions of soil fauna: Collembola, Psocoptera, Diptera, Anellida and Diplopoda |
Dajoz ([18]) highlighted how European beech and silver fir reveal a progressive increase in the biomass of insects, when wood decomposition reaches decay stages 1-3. Moreover, the relative number of predators (xylophagous), the specification and the index of diversity wealth also rise, with higher presence of insect-plant specialization.
Colonization and succession patterns of invertebrate communities
Knowledge on the saproxylic invertebrate communities occurring in the different stages of decay is mostly based on qualitative and descriptive studies. There are few quantitative analyses that have investigated the wood decay process, in relation to the composition of invertebrate communities ([80]). Different taxonomic groups attack weakened trees, such the subfamily Scolytinae (Curculionidae). The different species of this family can colonize both the moribund and the dead trees.
Moribund trees. Scolytinae represent the first subfamily of insects that attack the weakened trees. They are able to identify host trees by the presence of volatile compounds, such as monoterpenes, released by dying trees ([13]). The first individuals colonizing the moribund trees release aggregation pheromones that attract other individuals of the same species. This may start a mass attack where thousands of bark beetles carry the tree to exhaust the resin defences ([72]). The beetles bring with them also many species of fungi that contribute to the tree death. Afterwards, the tree becomes susceptible to the colonization by other saproxylic invertebrates.
Dead trees. Once the tree is dead, it can be colonized by other species. For example, among the 84 species of Scolytinae occurring in Sweden, 14 attack weakened trees, while 70 species use recently dead trees ([22]). Scolytinae complete their wood galleries in a couple of weeks, often accompanied or followed by other insects that feed on the inner bark, such as some species of Cerambycidae and Curculionidae. Then, the larvae dig their tunnels consuming the inner bark.
Major European forest tree species (e.g., Picea abies, Abies alba, Fagus sylvatica, Pinus sylvestris) generally reveal a community of 50-150 species of insects, including predators, such as Diptera and Hymenoptera parasitoids. Kenis et al. ([45]) listed 115 species of insects, beetles and Diptera, as potential predators of Scolytinae. Some species of predatory beetles live in the galleries made by Scolytinae, including various species of Histeridae. They are associated with other families, such as Rhizophagidae, Trogossitidae, Cleridae, Nitidulidae, Staphylinidae, Tenebrionidae. However, many of these species are probably fungivorous and may only act optionally as predators or scavengers. Moreover, Kenis et al. ([46]) listed 175 species of Hymenoptera parasitoids of different species of European bark beetles. Many species are specific parasitoids. Some of them are polyphagous and attack various Scolytinae in different tree species, while others are associated to a single host species. Braconidae, Pteromalidae and Eurytomidae can be considered the most important parasitoids of Scolytinae.
Some larvae of beetles (Cerambycidae and Curculionidae), characterized by subcortical development, are usually larger than the larvae of Scolytinae and, therefore, often affected by other species of parasitoids ([45]).
Subcortical zone and first decay stage
In differentiating the succession of saproxylics organisms across the decay stages, we considered the classification system developed by Dajoz ([18]), as being widely used in literature.
The subcortical area of the tree trunk is affected by the action of species that feed on phloem and are often associated with fungi that grow between the bark and the wood surface ([91]). The species of this group do not build recognizable galleries or tunnels but move freely below the bark. These species have their predators and parasitoids other than those associated with bark tissues ([80]). Many species of beetles at this stage of succession have a specific adaptation to the subcortical area, occurring both as larvae and adults ([14]). The beetle families living in these conditions are the larvae of Pyrochroidae and Pythidae. Various Cucujidae are also typical of the subcortical area in a dying tree. Moreover, a large variety of Diptera species belonging to many families can be found in the first stage of wood decay, including the Lonchaeidae, Dolichopodidae, Xylomyidae and Xylophagidae ([91]), among the others.
During the first stage of decomposition, the saproxylic community is dominated by primary saproxylics. They include pioneer organisms that colonize the intact or weakened trees, but also the recently dead trees ([89]). These species include beetles with strong jaws that allow them to dig tunnels in the bark and into the wood. They are able to digest cellulose, even specialized for metabolizing the cellular content and sapwood located under the bark, despite the presence of chemical defence still active in the wood ([80]).
Other primary saproxylics are the wood-eating mites (and fungi that are transported by some beetles - [89]). Among them, the adult phases of bark beetles and Platypodinae have specialized anatomical structures useful to contain the spores of fungi and micangi. This feature makes the saproxylic insects potential vectors of load for fungal infections to the tissues of the plant, also digging tunnels, dispersing debris and spreading fertilizer. In this phase, they determine the increase of the exposed wood surface to the external environment, also creating the “culture medium” for the development of mycelia. Many primary saproxylic beetles associated with coniferous species colonize trees subjected to physiological stress or illness, especially when the mechanism for the resin production is insufficient, so they can penetrate the bark and dig tunnels in the transmission layer without any resistance. Moreover, a crucial role is also carried out by the bark beetles, even if they constitute only a small portion of the primary saproxylic invertebrates of the European fauna, representing, however, an important amount in terms of biomass ([14]). They are distinguished from other primary saproxylic organisms because they do not possess the enzymes cellulase and hemicellulase, which should allow them to digest part of the cell wall of woody tissues ([89]). As a result, their activity is concentrated in the transition area, just below the bark, where the widest variety of mobile content is available as food ([89]). Most of the bark beetles carries symbiotic fungi that propagate through the wood, thus guaranteeing a supply of material for the beetle larvae; in some cases, the larvae use as a food source the same fungal spores.
Another group of beetles, weevils (Platypodinae) feed on the xylem tissues. These beetles have compensated their digestive limitations due to symbiotic fungi, transported micangi located on the cuticle, which can digest the wood more quickly ([91]).
Second stage of wood decay
At this stage, the subcortical habitat disappears and bark falls, and the fungal activity begins: it is characterized by the occurrence of the mycofagous insects that feed on the fruit bodies or mycelia developing in woody tissues ([92]). When the first fungi begin to produce their fruiting bodies, the number of fungivorous invertebrates rapidly increases. The main decomposer fungus is usually a polypore, able to accommodate different types of beetles. The larvae of beetles (Cerambycidae, Lucanidae and Trogossitidae) are very efficient decomposers that promote the physical disgregation. In the dry wood, the larvae of Buprestidae and Anobiidae are generally dominant. Furthermore, the percentage of mycofagous insects very often increases in relation to the species of wood-eating detritivores; they generally anticipate the real decay process.
The secondary saproxylics are represented by all those species that use the woody tissues partially degraded by the primary saproxylics. The second phase occurring in the wood decay process is characterized by the partial bark detachment. This phase can be found in dead trees and portions of them (trunks and branches, as well as coarse woody debris and stumps), but also on senescent trees ([91]). Insects belonging to this phase are characterized by larvae with special anatomical and physiological adaptations, such as hard seed coats, strong mandibles and possessing a digestive system that can metabolise the wood components. Numerous species of beetles, such as Cerambycidae and Buprestidae ([75]), are representative of this stage of decay. Moreover, also the family of Lucanidae includes species at the larval stage that occur on dying trees or rotting logs and stumps. Yet, beetles referring to the families of Melandrydae, Scarabeidae, Curculionidae and Bostrichidae also occur.
Furthermore, after the mechanical damages arising on the woody tissues (often induced by the atmospheric agents, by the xylophagous insects, or following the attack of fungi), suitable microhabitats appear in the whole woody structures. They are a good substrate for the development of the larval stage of Diptera, which can be considered as secondary saproxylic species ([75]).
Moreover, in the second stage of decay, species belonging to the order of the Hymenoptera also appear, as well as the families of Siricidae and Xiphydriidae. Their larvae feed on xylem tissues, while some species of Formicidae, digging nests in dead trunks, contribute to the degradation of deadwood. Some species of wood-eating insects prefer the dry wood, often exposed to the direct sunlight. Among these, many species belong to Buprestidae (Coleoptera), usually growing in the middle and upper parts of trees (e.g., European beech), or also on standing dead trees (e.g., Norway spruce), begin to disgregate deadwood ([25]). Moreover, longhorn beetles are also associated to deadwood of deciduous broadleaves (e.g., European beech) or conifer species (e.g., silver fir, Norway spruce - [14]).
Third and fourth stages of wood decay
As the decomposition process increases, the number of primary saproxylic invertebrates grows together with their associated predators ([97]).
At these stages, most of the sapwood is strongly fragmented and also the heartwood begins to decay. Few insects feed on the wood, still associated with their predators. Some species of beetles that usually colonize the wood at this stage of decay can perform several generations until the wood is completely consumed ([91]). The Elateridae are another group of beetles that colonize the wood in the advanced stages of decay. These species are slowly being replaced by the litter invertebrates, such as molluscs. Many beetles, such as Carabidae, use this microhabitat for their hibernation and aestivation. Secondary saproxylics that use partially decomposed wood persist, while food availability diminishes. At this stage, the wood is transformed into a reddish-brown friable mass, largely composed of the faeces of saproxylics ([97]).
In the advanced stages of decay, the deadwood loses its physical structure, including heartwood. In these conditions, the wood is almost completely consumed and it appears as a weakened mass composed in large part by the excavation of tunnels and secondary organic products, deriving from the droppings of saproxylics ([91]). The typical wood species occurring in this state of decomposition overlap with those typically present in the litter. In addition to fungi, the most common taxa observable in this phase are mainly the following: springtails, beetles, Diptera, isopods, Centipedes, annelids, nematodes, mites, pseudoscorpions and gastropods ([76]). Many of these organisms are feeders, predators, mycophagous, detritivores and their action on the substrate, together with the action carried out by bacteria, determines the humification of the woody organic matter, the last phase of the wood decay chain.
The microorganisms occurring in this phase are mainly microphages that feed on tertiary saproxylics, bacteria and micro-fungi, degrading the wood towards the final stage of humification of its decomposition.
The trunk, therefore, turns into a bedding that crumbles in a few years ([80]). Obviously, the process of wood decomposition does not take place in such an orderly manner in time and space as it has been described. Many saproxylic species representative of the decomposition will be present together during the overall process ([80]).
Interspecific interactions
Many saproxylic species belonging to the Cerambycidae, Lucanidae or Cetoniidae families are recognized as ecosystem engineers ([12], [81]). They may coexist in different stages of wood decay, with their action allowing a wider range of woody microhabitats (ecological attributes) and richer saproxylic communities (community attributes). Interspecific interactions may model the saproxylic biodiversity.
Various species of beetles live in deadwood in combination with other saproxylics, usually being subcortical predators. Among the beetles, the Elateridae include predatory species, both at the larval and adult stage ([89]). Among the Cleridae, a predator group of insect species, some are specifically predators of wood-eating insects. The Cleridae are able to identify their prey by specific chemical interactions. Among the Histeridae (Coleoptera), many species are predators of eggs and larvae belonging to wood-eating insects. Moreover, the Zopheridae (Coleoptera) include taxa that predate larvae of Scolytinae and mites, but also other predators that typically live in the wood, e.g., of European beech trees ([25]). Wende et al. ([99]) observed remarkably high specialization of deadwood-feeding xylophagous beetles and lower specialization of fungivorous and predatory species in deadwood of 13 tree species in Europe.
Furthermore, among the Carabidae species, Curculionidae (subfamily Scolytinae) and Cerambycidae are elusive and predatory beetles ([89]). In addition, among the Cucujdae, the polyphagous species are predominantly predator that feed both larvae and adults of saproxylic invertebrates, as well as the tree phloem and organic substances of animals, plants and fungi.
Other groups of insects are also predators, like some saproxylic Diptera Xylomyidae and Sciomyzidae ([89]). Many wasps are parasites, parasitoids or hyperparasitoids of saproxylic insects; among them, the following groups are frequent: Orussidae, Stephanidae, Aulacidae, Chalcidoidea and parasitic wasps. Finally, some insects use deadwood as shelter or for overwintering ([89]).
Saproxylic fungal communities
Other key elements of the biodiversity occurring in deadwood are represented by the saproxylic fungi ([91]).
Saproxylic fungi, or “wood-inhabiting fungi”, often used as a synonym, play a crucial role in deadwood decomposition and nutrient cycling. Moreover, these fungi are directly responsible for the generation of new habitats and food resources for other wood-dependent organisms ([9]), especially insects, as discussed in the previous chapter. Therefore, fungi represent a fundamental element for the functioning of forest ecosystems, in particular, where resource availability is guaranteed by natural disturbance dynamics.
The high efficiency of saproxylic fungi in decaying woody substrates is due to the production of extracellular enzymes involved in the decomposition of recalcitrant compounds, such as lignin ([2]). However, not all the species are able to accomplish a complete degradation of wood. According to the enzymes they produce, decomposer fungi are commonly classified as white-rot, brown-rot and soft-rot fungi. The latter group creates cavities by decomposing cellulose and hemicellulose and it is also involved in the degradation of lignin. Brown-rot fungi mainly degrade cellulose and hemi-cellulose, while their capacity to alter lignin is limited. White-rot fungi are capable of degrading all the major polymers, often prevailing in the final stage of wood degradation ([73] and references therein). As a consequence, deadwood decomposition results in a heterogeneous process carried out by a mosaic of fungal species with different decomposition abilities and ecological specialization ([9]).
Deadwood decay rates are influenced by the variation of the microclimatic conditions, but also by the fungal species composition and the related interactions among species. Furthermore, the species immigration history may also have a role in regulating the wood decay process and the carbon release. In this context, historical influences of community assembly should be included into models for carbon dynamics to achieve more reliable predictions of ecosystem functioning.
In fungal ecology, it is a common thought that the increase in species richness results in the enhancement of nutrient cycling and decomposition via additive or synergistic activities ([95]). Conversely, a reduction of biodiversity may negatively affect the decomposition rates in a given forest environment ([38]). However, very few studies reported a clear effect of species-richness on wood decomposition rates. Positive effects of fungal diversity on the decomposition processes have been observed in microcosms experiments with relative few species ([85]). Nonetheless, when the species richness is further increased, no clear patterns have been reported, suggesting a rapid niche saturation ([58]). In this case, competitive interactions for space may prevail, resulting in a reduction of metabolic energy available for decomposition ([95]). In a study on polypore fruiting bodies, Jacobs & Work ([37]) found that, in field conditions, the overall composition of fungal communities is not determinant for explaining the variations in decay rates. However, focusing only on six dominant species, they might have underestimated the total fungal diversity. Another study conducted by Hanson et al. ([32]) provided evidence for resource partitioning among soil fungi within a laboratory setting, emphasizing the need to prove the same process within natural ecosystems. If decomposer organisms do not equally utilize resources, then relatively high taxonomic diversity may be relevant for maintaining carbon and nutrient turnover in forests. The rate of mass loss in old stumps has been linked to specific traits of fungal species, supporting the hypothesis that the presence or absence of a species with marked decay abilities, and the interactions between species during community development, may affect the decomposition processes ([96]).
In conclusion, no clear relationships between decomposition rates and decomposer diversity have been demonstrated so far. The effect of fungal species richness on the decay rates has not been extensively verified in situ and more experiments under different environmental conditions are needed ([73]). The type of interactions involved, the presence of species with key functions and the composition of the organic resources, should not be neglected when investigating the nature of the relationship between community structure of fungal decomposers, resource quality and decay rates ([95]).
Saproxylic fungi and their relationship with the substrate characteristics
The substrate quality, associated with the microclimatic conditions, are likely the main factors influencing the abundance and diversity of fungi in decaying wood ([4]). Understanding the utilization of resources by different fungal taxa in relation to the host species, size of woody substrates and decomposition stage, may reveal important suggestions for conservation purposes.
In the following sections, we report the characteristics of deadwood that may affect the diversity and composition of saproxylic fungal communities.
Decay stage
As seen for saproxyilic insects, the composition of fungal community changes as deadwood decay proceeds. Different species, such as Eutypa spinosa (Pers.) Tul. & C. Tul. and Fomes fomentarius (L.) Fr., are known to be latently present in the sapwood as endophytes in (living) European beech branches, switching to pioneers as soon as the micro-environmental conditions become suitable ([64]). These early colonizers are mainly non-decaying taxa, but they may utilize the resources, with specific ecological requirements ([39]), before the ingression of early secondary and late secondary colonizers, which can be stronger competitors ([70]). Some examples on functional groups and successional patterns are given in Tab. 2 and Fig. 1. As a result, logs in various stages of decay differ in their species composition.
Tab. 2 - Main characteristics of fungal groups succeeding on deadwood from fresh to highly decomposed (modified from [39]).
Successional group | Characteristics | Main taxa |
---|---|---|
Early colonizers | Opportunists. The colonization could start as airborne spores, latent endophytes or pathogens adapted to inhabit living tissues. | Non-decaying species |
Early secondary colonizers | Intermediate competitive abilities. | Wood- decaying species increase |
Late secondary colonizers | Strong competitive abilities. Replace the majority of the pioneers. Able to degrade recalcitrant compounds. Usually the colonization results from spore dispersal or mycelium growth. | Mainly wood-decaying basidiomycetes |
Fig. 1 - (A) Example of a senescent tree and the variety of microhabitats it may provide to saproxylic species. (1): polypore fruiting bodies; (2): open wound; (3): dead root; (4): basal cavity; (5): dead sun-exposed branch; (6): branch cavity; (7): trunk cavity (modified from [91]). The figure can also be seen in terms of saproxylic species succession. Early colonizers may establish in living and weakened trees. These pioneers are going to be replaced by other more competitive species when a branch falls on the forest floor (8a). As decay proceeds (8b), secondary and late colonizers appear in the succession. (B) Examples of saproxylic species of insects and fungi succeeding during wood decomposition (pictures not in scale - drawings by S. Pioli).
Recent studies based on fruit body surveys have confirmed the hypothesis that saproxylic fungi have different growing patterns depending on specific deadwood properties, including the decomposition stage ([1]). According to these results, fungal taxa showing a preference for wood in a certain stage of decomposition can be classified as decay stage specialists. On the other hand, those species able to colonize coarse woody debris at any stage of decay are considered generalists. A similar pattern has been reported for preferences regarding wood diameter classes, but this feature is further investigated in the “Diameter” section of this chapter. Certain fungal taxa are known to show a marked preference for specific decay classes. In particular, species such as Hypoxylon fragiforme (Pers.) J. Kickx f. and Lopadostoma turgidum (Pers.) Traverso, were found almost exclusively on fresh wood, while Trechispora farinacea (Pers.) Liberta and Phanerochaete velutina (DC.) P. Karst. occurred more frequently in the higher stages of decay ([7]).
It has been suggested that well decayed deadwood hosts a higher variety of microhabitats caused by heterogeneous decomposition, therefore, supporting a more diverse fungal community. Higher number of species were found in more advanced decomposition stages by studies comparing fruit bodies inventories and molecular approaches ([24]). Furthermore, logs in advanced decay stages have been reported to be especially important for red-listed species, considered also threatened at regional scale ([6]).
Deadwood type
The diversity of the substrates in terms of deadwood types, like trunks, branches and twigs, stumps and snags, must be taken into account when studying the saproxylic fungal richness ([7]). Few studies compared the species richness and composition on logs and stumps; however, the latter were reported to host a poorer community with respect to lying dead logs, especially on Norway spruce ([52], [6], [7]). The proportion of unique species and the number of rare species are commonly higher on logs, revealing a more diverse community, when compared with the stumps-inhabiting assemblages. An explanation for these findings could be related to the different decay rates between logs and stumps. Indeed, deadwood lying on the forest floor has a higher surface in contact with the soil moisture, which may influence the rates of decomposition. However, there are contradictory statements in the literature related to this issue. Lindhe et al. ([52]) reported that the higher wood moisture in logs may lead to a faster decomposition as compared to stumps, thus allowing more species to develop during a certain time lapse. On the other hand, the higher density of wood in stumps may also play a role in influencing moisture retention and decomposition rates; therefore, in some cases, stumps decompose even faster compared to logs ([86]).
Besides the moisture content and the wood density, other important factors explaining the differences in species composition between stumps and logs are linked to the colonization patterns. In stumps, the presence of an exposed cut surface area and the root system in contact with the ground, may facilitate the fungal colonization through airborne spores and mycelia from the soil, respectively ([6]).
Notwithstanding stumps do not probably support rare and threatened species, due to their highly specialized niche requirements ([21]), they may have a role in maintaining species richness in managed forests, where often stumps are the only occurring deadwood component ([94]) (Fig. 2).
Fig. 2 - Main differences of logs versus stumps and characteristics of the associated fungal community.
In much of the existing literature, snags are described as the most species-poor deadwood type ([69], [86]), probably because of the unstable microclimatic conditions and the lower habitat availability in comparison with downed logs and stumps.
Host tree species
Host tree species is widely considered another important factor influencing the distribution patterns and the species richness of decomposing fungi ([48], [43]). Host preferences is likely related to some fungal groups that are specialized on specific trees; this is particularly evident when comparing deciduous broadleaves and coniferous trees, in which the fungal composition is clearly different ([7]). For example, Norway spruce and Scots pine host more red-listed polypores and corticioids than deciduous hardwood species in Finland ([59]). The importance of tree species heterogeneity to increase the fungal diversity in temperate forests has often been pointed out, especially for the corticioid basidiomycetes group ([48]). However, the richness of the tree layer has not been always considered a useful indicator for predicting fungal species richness ([79]).
Debris size
The majority of the studies on saproxylic organisms have so far focused on CWD, since larger deadwood components have been more often removed by forest management practices ([87]), but also because of practical aspects related to the fruit body surveys ([41]). However, a comprehensive analysis of fungal richness and community diversity should include smaller diameter debris as well ([7]). Small deadwood pieces are characterized by a higher surface-to-volume ratio that facilitates the fungal colonization ([35]). On the other hand, debris of larger diameter persists longer on the forest floor due to the slower decay, allowing the coexistence and succession of a higher number of species characterized by different ecological requirements. Moreover, larger and older logs may reflect a longer infection history of certain specialized heart-rot pathogens inhabiting living sapwood ([61]). Furthermore, not only the colonization, but also the extinction patterns can be affected by the deadwood size, as local extinction rates increase on logs with small diameter compared to the larger ones ([39]). Different species are diversely influenced by diameters. For instance, common species seem to have little preferences for certain wood dimensions ([52]), while some threatened or endangered species occur more frequently on larger substrates, often typical of old-growth forests. In general, all those species that depend on stable microclimatic conditions prefer larger trunks, in which these conditions often occur.
Many authors reported a positive relationship between CWD size and saproxylic species richness ([35], [52]). Bässler et al. ([4]) and Pouska et al. ([69]) revealed that the number of threatened and specialized species rises with increasing the mean diameter of logs in mountain spruce-dominated forests. A more recent study ([1]) has suggested that some species or families occur preferably on a certain substrate diameter; in particular, Polyporaceae and Botryobasidiaceae are two of the families classified as size specialists. On the other hand, Rajala et al. ([74]), in a study on Norway spruce logs, did not report any significant relationship between species richness and log diameter, although the community composition was affected by log volume and diameter.
Fine woody debris can also be important for the occurrence of certain taxa ([94]) and for the total species richness of saproxylic fungi in a given forest stand. Juutilainen et al. ([41]) studied the effect of excluding the finest fractions of deadwood (less than 1 cm diameter) from mycological surveys. The authors reported an underestimation of the species richness by 10% and occurrences by 46% when the finest debris is not considered for fruit body occurrences of polypores and corticioid fungi. Moreover, species assemblages differ noticeably among diameter classes, supporting the hypothesis that fine and very fine woody debris are important microhabitats for specific communities. Similar results were achieved by earlier studies in temperate broadleaf, deciduous and conifer forests ([48]). Fine and very fine debris play an important role for maintaining the overall diversity of saproxylic fungi, especially for the occurrence of common species. In some cases, rare species occur on very fine woody debris more frequently than on CWD, suggesting that at least some of the species considered endangered or red listed are rarely observed on finest fractions, since sampling effort has been mostly focused so far on CWD ([42]). In Tab. 3 some details on the effects of deadwood diameter on decomposer fungal communities are reported.
Tab. 3 - Observed effects of deadwood diameter over fungal community richness and composition across different forest types in mountain environments.
Target group | Forest type | Effect | Source |
---|---|---|---|
Wood-inhabiting fungi | Norway spruce | Species composition differed according to log size | Edman et al. ([21]) |
Wood-inhabiting fungi | European beech | Increasing species diversity per volume unit with decreasing log size | Heilmann-Clausen & Christensen ([35]) |
Ascomycetes and Basidiomycetes | Spruce, birch, aspen and oak | Mean species number is positively related to substrate diameter | Lindhe et al. ([52]) |
Wood-inhabiting fungi | European beech, Norway spruce, fir, Scots pine, sweet chestnut | Different species have different preferences for wood size | Küffer & Senn-Irlet ([47]) |
Polyporaceae and Corticiaceae | Norway spruce | Higher extinction rates on small-diameter logs | Jönsson et al. ([39]) |
Basidiomycetes and ascomycetes | Fagus crenata | No significant effect | Fukasawa et al. ([28]) |
Pioneer wood-inhabiting fungi | Norway spruce | Occurrence probability of the species increased with increasing diameter | Berglund et al. ([6]) |
Polypores and corticoids | Pine and spruce | Species number increase with increasing diameter class | Stokland & Larsson ([90]) |
Wood-inhabiting fungi | Beech | Different fungal groups have different growing patterns according to diameter | Abrego & Salcedo ([1]) |
Wood-inhabiting fungi | Beech and conifer | Larger diameter items hosted more species | Blaser et al. ([7]) |
Saprotrophic fungi | Pinus densiflora | Community composition is positively related with log diameter |
Fukasawa & Matsuoka ([27]) |
Saproxylic fungi as indicators of sustainable forest management
Communities of saproxylic fungi are very sensitive to changes in forest structures: they may alter the occurrence of microhabitats and their characteristics. For this reason, there has been an increased interest in recent literature on the effects of silvicultural practices that may preserve key habitats and enhance fungal diversity ([31], [20]).
Many studies focused on mountain forests, as these environments were managed with varying intensity of thinning during the past centuries. An interesting study by Bässler et al. ([5]) compared mountain spruce forests subjected to three different management regimes: formerly managed, disturbed by bark beetle activity and old-growth forests. They found more red-listed species in old-growth stands compared to the other study areas, suggesting to implement the network of protected areas to reduce the old-growth patches isolation. These authors also stressed the importance of structural complexity and deadwood variety as two of the main factors that may enhance the saproxylic fungal diversity. In Mediterranean mountain forests, the positive effect of increased stand structural heterogeneity on fruit body abundances and species diversity of wood-inhabiting fungi was also proved ([67]).
Improving disturbance-based forestry systems to maintain saproxylic fungal diversity has been recently considered as a promising practice in forest management ([20]). The observed increase of species richness could be partly due to the retention of CWD as important substrate for these fungi, but also the high level of aboveground biomass and the spatial variability in canopy closure played a role in fungal growth. The effect of canopy gap creation over decomposer fungal abundance and diversity has been previously tested alone and in combination with CWD addition by Brazee et al. ([11]). In the first case, no significant reduction in fungal abundance was reported, but when CWD creation was included, species abundance and diversity consistently improved.
Forest management directly affects the variety, volume, and quality of deadwood ([1], [7]) and recommendations are needed to preserve the fungal diversity and forest functioning. Management plans aimed to mimic natural disturbances seem to have a positive effect on the abundance and diversity of saproxylic fungi, as well as on the resistance and resilience of the whole forest ecosystem ([84]). These practices include, for example, the retention of woody debris of all size, types and decay classes comparable to those characterizing unmanaged and old-growth forests ([11]). In some cases, besides the retention of natural downed wood, it is worth creating new substrate by felling or girdling trees, especially if the aim is the implementation of forest reserve network ([7]).
The effect of management history and specific silvicultural practices over fungal communities have been widely debated in the literature, often with contrasting results according to the type of treatments, the methodological approaches and the target species investigated (Tab. 4). Interestingly, Halme et al. ([31]) reported a stronger effect of forest management on fungal communities specialized in the more advanced wood decay stages, suggesting that the consequences of silvicultural treatments require long time to be detectable.
Tab. 4 - Examples of different silvicultural treatments and their effect over specific groups of decomposer fungi.
Treatment | Type of effect | Target group | Source |
---|---|---|---|
Structural complexity enhancement | Increase sporocarp diversity | Macrofungi | Dove & Keeton ([20]) |
Decaying wood restoration | Increased average number of common species | Wood-decaying polypores | Pasanen et al. ([66]) |
Canopy gap creation | Change in community composition. No significant reduction in the abundance and diversity | Wood-inhabiting fungi | Brazee et al. ([11]) |
Partial cutting | No significant effect | Pioneer wood-inhabiting fungi and saproxylic fungi | Berglund et al. ([6]) |
Wood harvesting | Reduction in polypore occurrences and in total number of fungal taxa | Polypores, saprotrophic agarics and pleurotoid agarics | Toivanen et al. ([94]) |
Controlled burning | Change in community composition | Pioneer wood-inhabiting fungi | Berglund et al. ([6]) |
Forest conversion (e.g., from beech to conifer species) |
Changes in community structure. Significant reduction in fungal OTU richness and diversity | Wood-inhabiting fungi | Purahong et al. ([71]) |
Stand-replacing wildfires |
Decreased species richness. Altered species composition | Decaying fungi | (Kurth et al. ([49]) |
The interaction of the different abiotic factors illustrated in Fig. 3 can be considered responsible for driving the fungal (and insect) community structure in terms of species richness, composition and dynamics, therefore affecting the decay patterns and, consequently, the forest ecosystem functioning.
Fig. 3 - Main abiotic factors and their interactions that influence saproxylic community diversity and composition.
Saproxylic-oriented forest management
The study of saproxylic communities, their interactions and dynamics is a powerful tool for inferring the decomposition patterns and carbon sequestration capacity of forest environments under future climatic scenarios. However, the need for preserving specific ecosystem functions is often hindered by the practical constraints of forest management that have led to simplified biological communities in many forest systems across forest ecosystems in Europe. The future challenge for forest managers will be to find a balance between the conservation of biological diversity and the sustainable use of woody resources. To this purpose, we herewith reviewed the requirements of saproxylic species regarding resource utilization and environmental conditions.
Amount, distribution, average size and decay stage of deadwood affect the saproxylic communities that require a higher variety of niches for supporting the differently specialized assemblages. However, a deeper understanding of threatened population dynamics must be taken into account when specific management targets need to be addressed. Red-listed species are known to have significant habitat preferences ([35]) and more studies should focus on their occurrence patterns, particularly in mountain forests. Moreover, species that depend on rare substrates and/or species with limited colonization ability are particularly affected by silvicultural practices that reduce habitat continuity.
Fungal succession during the deadwood decay processes and the interaction with specialized saproxylic fauna represent an important issue for ecosystem functioning in forests. For this reason, the presence of natural decaying logs with different degrees of decomposition must be regarded as key elements for an efficient biodiversity-oriented management ([8]).
Specifically, mountain forests in Europe, as well as in other temperate climates, have been subjected to anthropic disturbances for centuries (e.g., deforestation, fires) and have been recently facing other types of human-induced alteration, such as air pollution, soil acidification and increased extreme climatic conditions (e.g., drought spells, heat waves). It is noteworthy that the predicted increase in extreme disturbance events like windstorms, will affect microclimatic conditions which, in turn, influence the community composition of saproxylic species. However, these aspects have been poorly investigated so far and deserve further efforts.
We stress the need for the preservation and valorisation of mountain forests through a responsible management that takes into account the conservation of saproxylic diversity and their habitat, both at local and regional scales. The functionality and productivity of montane ecosystems should be achieved by improving the structural heterogeneity and through an accurate assessment of deadwood patterns across spatial and temporal scales. The importance of deadwood on biodiversity conservation of forest ecosystems is widely recognised. Recent studies have confirmed its role in maintaining species diversity, increasing carbon content and enhancing biological activity in the soil ([8]).
Past management focused on what was being extracted from the forest, whereas current management emphasizes what is left behind. In this context, quantity and quality of CWD are regarded as important structural indicators of naturalness and biodiversity ([40]), providing information on the intensity of past human disturbances and closeness to old-growth forest condition ([100]). The needs to provide continuity to timber production and the requirements for favoring the presence of deadwood, however, are not in contrast with each other. It is certainly possible to use both the logs of good quality and easily accessible and leave deadwood on the forest floor, as well as trees damaged or of poor quality, old, uprooted, or dead standing. In order to increase the diversity of species of saproxylic organisms, mixed forest stands, as well as diversified stand and age structures should be encouraged, taking in account the degree of deadwood decomposition and the penetration of solar radiation. Good solar radiation is, for example, important for several species of Buprestidae and Cerambycidae. Besides deadwood, the development of various larvae also requires the existence of other sources of nourishment, such as pollen and plants whose presence is greater in forest with open canopy gaps or near forest edges. These structures and forest substrates should be available at all times and be well distributed within forest stands.
The significantly increasing demand for renewable energy resources, such as fuelwood - to reduce dependence on fossil fuels and thereby limit greenhouse gas emissions - may drive an intensification of forest management in the coming decades. Consequently, the amount of large over-mature trees and deadwood could be reduced. Such an intensification of management is expected to have a negative effect on saproxylic biodiversity ([78]). However, with increasing awareness towards the conservation of biodiversity, an intensification of forest management can still be possible with sustainable and adaptive forest management approaches, and/or through “land sparing” strategy, thus increasing forest yields on productive forests and reducing pressure on high nature value forests. This can only be achieved with the support of all the forest actors, including forest researchers, forest owners and decision makers.
There is a growing evidence that the rate of warming is amplified with elevation, such that high-mountain environments experience more rapid changes in temperature than environments at lower elevations. Elevation-dependent warming can accelerate the rate of change in mountain ecosystems, cryospheric systems, hydrological regimes and biodiversity ([56]). Increasing temperature at high elevation may have contrasting effects on biogeochemical processes, not necessarily associated with an increase in nutrient cycling and nutrient availability. It is essential to understand and quantify the role of saproxylic species and the relative importance of insects and fungi, thus assessing temporal and spatial changes in deadwood with varying elevation, particularly in Mediterranean environment, as they relate to forest regeneration, fuel load and fire risk ([78]).
Saproxylic organisms provide an important ecosystem service, decomposing organic matter and cycling the soil nutrients. However, it is still not well understood how sensitive is this service to the loss of saproxylic biodiversity. The complex interactions in the saproxylic food web suggest that the loss of basic decomposers (representing specific decay pathways), as well as cascading effects on higher trophic levels, may influence key ecosystem processes. This calls for studies on the relations between the decomposer species, including studies on competition, facilitation and other kinds of species interactions, in relationship with tree species diversity. We encourage forest managers to become aware of the importance of maintaining the diversity of organisms decaying wood jointly with the promotion of high tree species diversity (Fig. 4).
Fig. 4 - Adaptive management scheme. Input from research to provide knowledge synthesis, suggest modelling approaches, identify future targets, and design and evaluate monitoring schemes (modified from [98]).
General management rules can be envisaged to foster the biodiversity of saproxylic insects and fungi in mountain forest ecosystems ([51], [57]): (a) maintain ecological connectivity; (b) preserve the integrity of associated water systems, supporting hydrogeological and geomorphological processes; (c) promote stand structural complexity; (d) increase the heterogeneity of the landscape and the establishment of “senescence islands” in the forestry sector; (e) exploit the regime of natural disturbances to guide management in forests; (f) preserve the remaining areas of natural forests, favoring their heterogeneity and ensuring the maintenance of abundant woody materials on the forest floor; (g) ensure the presence of larger trees and, possibly, artificially accelerate the natural processes of constitution of suitable reproductive sites for saproxylic organisms.
Acknowledgements
This study generated from the COST (European Cooperation in Science and Technology) Action CLIMO (Climate-Smart Forestry in Mountain Regions - CA15226) financially supported by the EU Framework Programme for Research and Innovation HORIZON 2020.
FP and SP have contributed in equal measure to the writing of this article.
References
CrossRef | Gscholar
Gscholar
Gscholar
Gscholar
Gscholar
Gscholar
Gscholar
CrossRef | Gscholar
CrossRef | Gscholar
CrossRef | Gscholar
Gscholar
Gscholar
Gscholar
CrossRef | Gscholar
Gscholar
Authors’ Info
Authors’ Affiliation
Roberto Tognetti
Dipartimento Agricoltura, Ambiente e Alimenti, Università degli Studi del Molise, v. F. De Sanctis, I-86100 Campobasso (Italy)
Giulia Fravolini
Marco Marchetti
Dipartimento Bioscienze e Territorio, Università degli Studi del Molise, c.da Fonte Lappone, I-86090 Pesche, Isernia (Italy)
Libera Università di Bolzano, p.za Università 5, I-39100 Bolzano (Italy)
Roberto Tognetti
The EFI Project Centre on Mountain Forests (MOUNTFOR), Edmund Mach Foundation, v. E. Mach 1, I-38010 San Michele all’Adige, Trento (Italy)
Dipartimento di Agraria, Università Mediterranea di Reggio Calabria, loc. Feo di Vito, I-89122 Reggio Calabria (Italy)
Corresponding author
Paper Info
Citation
Parisi F, Pioli S, Lombardi F, Fravolini G, Marchetti M, Tognetti R (2018). Linking deadwood traits with saproxylic invertebrates and fungi in European forests - a review. iForest 11: 423-436. - doi: 10.3832/ifor2670-011
Academic Editor
Massimo Faccoli
Paper history
Received: Oct 27, 2017
Accepted: Apr 14, 2018
First online: Jun 18, 2018
Publication Date: Jun 30, 2018
Publication Time: 2.17 months
Copyright Information
© SISEF - The Italian Society of Silviculture and Forest Ecology 2018
Open Access
This article is distributed under the terms of the Creative Commons Attribution-Non Commercial 4.0 International (https://creativecommons.org/licenses/by-nc/4.0/), which permits unrestricted use, distribution, and reproduction in any medium, provided you give appropriate credit to the original author(s) and the source, provide a link to the Creative Commons license, and indicate if changes were made.
Web Metrics
Breakdown by View Type
Article Usage
Total Article Views: 21969
(from publication date up to now)
Breakdown by View Type
HTML Page Views: 16147
Abstract Page Views: 1432
PDF Downloads: 3600
Citation/Reference Downloads: 30
XML Downloads: 760
Web Metrics
Days since publication: 2231
Overall contacts: 21969
Avg. contacts per week: 68.93
Article Citations
Article citations are based on data periodically collected from the Clarivate Web of Science web site
(last update: Nov 2020)
Total number of cites (since 2018): 13
Average cites per year: 4.33
Publication Metrics
by Dimensions ©
Articles citing this article
List of the papers citing this article based on CrossRef Cited-by.
Related Contents
iForest Similar Articles
Research Articles
Stand structure and deadwood amount influences saproxylic fungal biodiversity in Mediterranean mountain unmanaged forests
vol. 9, pp. 115-124 (online: 08 September 2015)
Research Articles
Exposure elevation and forest structure predict the abundance of saproxylic beetles’ communities in mountain managed beech forests
vol. 16, pp. 155-164 (online: 08 June 2023)
Research Articles
Saproxylic beetles in non-intervention and coppice-with-standards restoration management in Meerdaal forest (Belgium): an exploratory analysis
vol. 9, pp. 536-545 (online: 25 March 2016)
Review Papers
The forest biodiversity artery: towards forest management for saproxylic conservation
vol. 9, pp. 205-216 (online: 26 October 2015)
Research Articles
Diversity of saproxylic beetle communities in chestnut agroforestry systems
vol. 13, pp. 456-465 (online: 07 October 2020)
Research Articles
Decomposition of Norway spruce and European larch coarse woody debris (CWD) in relation to different elevation and exposure in an Alpine setting
vol. 9, pp. 154-164 (online: 28 August 2015)
Research Articles
Distribution and habitat suitability of two rare saproxylic beetles in Croatia - a piece of puzzle missing for South-Eastern Europe
vol. 11, pp. 765-774 (online: 28 November 2018)
Research Articles
The Habitat-Trees experiment: using exotic tree species as new microhabitats for the native fauna
vol. 8, pp. 464-470 (online: 22 October 2014)
Short Communications
Culturable fungi associated with wood decay of Picea abies in subalpine forest soils: a field-mesocosm case study
vol. 11, pp. 781-785 (online: 28 November 2018)
Research Articles
Use of fallen dead trees by Japanese squirrels within cedar plantations in northeastern Japan
vol. 16, pp. 262-267 (online: 19 October 2023)
iForest Database Search
Google Scholar Search
Citing Articles
Search By Author
Search By Keywords
PubMed Search
Search By Author
Search By Keyword