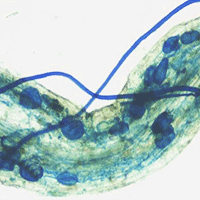
Arbuscular mycorrhizal colonization in black poplar roots after defoliation by a non-native and a native insect
iForest - Biogeosciences and Forestry, Volume 9, Issue 6, Pages 868-874 (2016)
doi: https://doi.org/10.3832/ifor1911-009
Published: Aug 29, 2016 - Copyright © 2016 SISEF
Research Articles
Abstract
A major goal in ecology is to understand how interactions among organisms influence ecosystem services. This work compares the effects of two Lepidoptera defoliators, one non-native (Hyphantria cunea) and one native (Lymantria dispar) to Europe, on the colonization of black poplar (the Populus nigra clone “Jean Pourtet”) roots by an arbuscular mycorrhizal (AM) symbiotic fungus (Funneliformis mosseae) in a pot experiment. The effects of defoliation have also been assessed on the expression of fungal and plant genes playing a role during symbiosis. Both control and defoliated poplars have shown a low level of mycorrhization. Additionally, neither the non-native nor the native insect seem to strongly affect the AM colonization, at least at the time of observation (eight days from the end of the defoliation). Concerning the gene expression analysis, our results suggest that defoliation does not influence neither the expression of genes coding for a fungal and a plant phosphate transporter nor that of a gene coding for a fungal ATPase, and that there were no differences between defoliation carried out by the non-native and the native insect.
Keywords
Exotic, Defoliators, Arbuscular Mycorrhizal Symbiosis, qPCR, Poplar, Gene Expression
Introduction
Terrestrial ecosystems can be roughly divided into above- and below-ground, linked together by plants ([46]). A major goal in ecology is to understand how interactions among organisms influence ecosystems ([6]). Invasive organisms - i.e. non-native organisms, which threaten ecosystems, habitat or indigenous species - are a major element of global change and are contributing to biodiversity loss, ecosystem degradation and impairment of ecosystem services worldwide ([1]).
More than 90% of plants form root interactions with mycorrhizal fungi that provide the host plant with water and mineral nutrients and can lead to an improved tolerance against biotic and abiotic stresses (e.g., salinity, drought, heavy metals), in exchange for photoassimilates ([39]). In particular, arbuscular mycorrhizal (AM) symbiosis involves fungi belonging to Glomeromycota ([37]) and the majority of land plants, including both important crop species, such as tomato, wheat, rice, maize, soybean, and forest tree species, such as poplars (Populus spp. - [40]). The root colonization process involves both epidermal and cortical cells, where the fungus develops inter- and intra-cellular hyphae, coils, and arbuscules ([17]). Despite the improved mineral nutrition, AM fungi cause a significant carbon cost for the host plant, allocating up to 20% of the photosynthates ([36]). AM fungi are obligate biotrophs and they are thus not able to survive long periods without the host plant ([4]). Enzymes for saprotrophic behavior are lacking in the genome of the AM fungus Rhizophagus irregularis, which has been recently sequenced ([41]). Over long-term, leaf herbivory (and clipping) may eventually result in reduced carbon accessibility for the host plant and fungal partners ([3], [36]), although, in spite of the re-growth of the above-ground tissue, carbon allocation to roots often increases immediately after defoliation, while allocation to shoots decreases ([11], [21]). Although the impact of leaf herbivory on colonization by mycorrhizal fungi has mainly been reported as negative, sometimes even positive or nil effects on fungal symbiosis have been detected ([15], [16], [10], [12], [20], [24], [32], [45], [14], [36], [42]). It has been reported that the variability in responses might depend on the type and extent of defoliation, the duration of the experiment, the mycorrhizal type or plant species considered, the availability of soil nutrients or other not yet known factors ([16], [14]). Barto & Rillig ([3]) conducted a meta-analysis study, focusing on the sensitivity of AM and ectomycorrhizal (ECM) colonization of roots to the removal of leaves or shoots by herbivory or clipping. Meta-analysis results showed that the carbon-limitation hypothesis of reduced mycorrhizal colonization following removal of above-ground biomass was generally not supported, and that herbivory (or clipping) did not reduce mycorrhizal colonization by biologically meaningful levels in many types of plants ([3]). More recently, Saravesi et al. ([36]) reported a different effect of host plant defoliation on root AM and dark septate endophyte (DSE) fungi colonization. In particular, negative impacts on AM colonization and a slightly positive impact on DSE colonization were observed, suggesting divergent ecological roles of the two fungal associates in the plant carbon economy.
The increasing threat of invasive species, among which are several defoliators, raises a question about their possible impact on the components of native ecosystems ([22]). In Europe, Hyphantria cunea Drury, a moth native to North America, became invasive in the 1940s ([47]). Being a polyphagous defoliator, it feeds on hundreds of host plants, including black poplars. P. nigra L. is also a host plant for native defoliators, such as Lymantria dispar L. In a scenario where losses of above-ground biomass might impact mycorrhizal response as previously reported ([16], [14]), the issue is whether invasive defoliators could play a role in affecting native ecosystem components such as AM fungi.
In this work we compare the effects of two Lepidoptera defoliators, a species non-native to Europe, H. cunea, and a native species, L. dispar, on black poplar (the P. nigra L. clone “Jean Pourtet”) roots colonized by the AM fungus Funneliformis mosseae (T.H. Nicolson & Gerd.) C. Walker & A. Schüßler 2010. In particular, we assessed the AM fungal colonization in the black poplar roots and the expression of fungal genes coding for a fungal amino acid permease (GmosAAP1), two H+-ATPases (GmHA5, GmPMA1) and a phosphate transporter (GmosPT), as well as of a plant PT gene (PopPT) which have a putative role during symbiosis. The effects of both partial and total defoliation by larvae of the two defoliators have been evaluated.
Material and methods
Mycorrhization of Populus nigra with Funneliformis mosseae
Cuttings of the P. nigra clone “Jean Pourtet” were harvested in January 2014 at the CREA-PLF (Casale Monferrato, Italy). They were kept in tap water for 5/7 days and then planted in pots (10 × 10 × 17 cm) containing quartz sand previously sterilized at 180 °C for 3h. For one month, they were watered twice a week with tap water in order to obtain shoots and roots.
Forty-five seedlings were then inoculated with Funneliformis mosseae BEG 12 (formerly Glomus mosseae) inoculum (MycAgro Lab, France), by mixing the inoculum with sterile quartz sand (30% v/v). All plants were watered three times a week with tap water, and kept in a greenhouse for approximately 75 days, following the natural photoperiod (from March to June 2014).
Defoliation of Populus nigra seedlings
The defoliation occurred between the 24th of June and the 16th of July 2014. The potted plants were kept in the field at Agripolis campus (Legnaro, Italy) inside cages to protect them from insect attack, under conditions that mimic the natural ones. Five theses were considered: undefoliated control plants, partial and total defoliation by H. cunea and partial and total defoliation by L. dispar. In the partial treatment defoliation, about 50% of the leaves was exposed to defoliation, whereas in the total treatment defoliation experiment the whole foliage was exposed to defoliation. To achieve this, the half-lower part of plant bearing about 50% of leaves was enclosed in the mesh bag in the partial defoliation thesis and the entire plant in the total defoliation thesis. Each thesis included nine replicates. Each plant had a single pot and was enclosed, kept separately from the others, in a mesh bag, where 5 larvae (fourth-fifth instars) of H. cunea or L. dispar were placed for partial defoliation and 10 larvae of H. cunea or L. dispar for total defoliation experiments, respectively. The plants were watered every two days and checked, evaluating the degree of defoliation and replacing any dead larvae. The experiment was stopped at the same time for all the plants.
Estimation of the mycorrhization
All plants were unrooted after eight days from the end of the defoliation treatment, in order to assess the effects of the pests on the mycorrhizal colonization. The whole root apparatus from each plant was washed under tap water and divided into two parts: one part was used to estimate the level of mycorrhiza formation (see below), while the other part was frozen in liquid nitrogen and kept at -80 °C for subsequent molecular analysis. Colonization was assessed using the method described by Trouvelot et al. ([43]). Twenty-cm-long root segments were sampled from each root system, cleared in 10% KOH at 45 °C for 1 h, washed twice in 10% KOH for 24 h, stained with 0.1% cotton blue in lactic acid ([27]), and mounted on slides.
Nucleic acid extraction and cDNA conversion
The DNA extraction was performed on about 100 mg of leaves and of roots using the DNeasy Plant Mini Kit® (Qiagen, Valencia, CA, USA) according to the manufacturer’s instructions.
The RNA was extracted from about 100 mg of roots using the “pine-tree method” ([8]). After extraction, RNA was cleaned of DNA, using Promega DNase (RQ1 RNase-Free DNase®, Promega Corp., Madison, WI, USA), and then measured using a NanoDrop® (ThermoScientific, Hudson, NH, USA). The absence of genomic DNA was verified through one-step retrotranscription PCR (One-Step RT-PCR, Qiagen) with primers (Gmostef1, Gmostef2) previously designed for the F. mosseae elongation factor reference gene ([9]). Briefly, the same extracted RNA was used as template for retro-transcription and for PCR amplification or for PCR amplification only (RT- reactions). The absence of signal after PCR amplification without retrotranscription was regarded as absence of DNA. Total RNA for each sample was used to synthesize the cDNA, according to the SuperScript II Reverse Transcriptase® (Invitrogen, Carlsbad, CA, USA) procedure using random primers.
Selected genes and primers
The fungal genes selected for the analysis were an amino acid permease (GmosAAP1 - [9]), a phosphate transporter (GmosPT - [2]) and two different H+-ATPases (GmHA5, GmPMA1 - [34]). The fungal reference gene was the elongation factor (Gmostef - [9]). Concerning the plant, an elongation factor 1, beta subunit (PopEF1) was used as reference gene ([33]), in addition to a phosphate transporter (PopPT) for which the primers were designed using the web-interface program Primer3Plus (⇒ http://www.bioinformatics.nl/cgi-bin/primer3plus/primer3plus.cgi/). To this aim, the mycorrhizal-inducible Solanum lycopersicum phosphate transporter 4 (LePT4 - accession AAV97730.2) was used to search the homologous in the genome of P. trichocarpa (⇒ http://phytozome.jgi.doe.gov/pz/portal.html#!info?alias=Org_Ptrichocarpa - [44]). Among the P. trichocarpa homologous sequences identified using the tomato LePT4 sequence, the Potri.015G022800.1 was the best hit. The poplar and tomato aminoacid sequences showed 76% of identity and 98% of coverage with 0.0 of E-value. In addition, the poplar aminoacid sequence showed 74% of identity and 98% of coverage with 0.0 of E-value with the high affinity inorganic phosphate transporter of Medicago truncatula (accession XP_013466381.1), which corresponds to the mycorrhiza-inducible MtPT4 (accession AAM76744.1).
The primers used in this work are listed in Tab. 1. Fungal primers were tested on poplar DNA in order to verify the absence of cross-amplification. The primers for PopPT were tested in silico to verify the amplification on the target gene. In addition, PCR fragment has been sequenced at BMR Genomics S.r.L. (Padua, Italy).
Tab. 1 - List of the primers used in the study and their temperature of annealing (Ta).
Name | Gene Function | Reference | Sequence | Ta (°C) |
---|---|---|---|---|
GmosPT For | Pi transporter | [2] | ACTGTTGGCGCTTAGTGCTTGG | 60 |
GmosPT Rev | CAGCCCAACTTGATTTTGGTACG | |||
GmPMA1F3 | H+-ATPase | [34] | CCAGTCAAGAAAGTTCCCAAG | 60 |
GmPMA1R1 | CACGTTCAGCTGCTGATATCG | |||
GmHA5up1 | AATAATGTCTCCGAGGCGAGG | 60 | ||
GmHA5up3 | TTGAAGTAGCTTGCCTTTTCGC | |||
Gmostef1 | Elongation factor | [9] | GCAGAACGTGAGCGTGGTAT | 63 |
Gmostef2 | ACCAGTACCGGCAGCAATAA | |||
GmosAAP1 | Amino acid permease | TACTCCTCCCACCGATTACG | 54 | |
GmosAAP2 | CCGATGATGAGATAGCCGAT | |||
PopPT4for | Poplar phosphate transporter |
This work | TACGCAACCTCAGGCGGATT | 60 |
PopPT4rev | CGGTTTCGGGCATCTTCATC | |||
EF1 F | Poplar elongation factor 1, beta subunit | [33] | AAGCCATGGGATGATGAGAC | 60 |
EF1 R | ACTGGAGCCAATTTTGATGC |
Relative fungal DNA abundance
To evaluate the relative fungal abundance in poplar roots, quantitative PCR (qPCR) was carried out with Connect™ Real-Time PCR Detection System (Bio-Rad Laboratories, Hercules, CA, USA). Each PCR reaction was conducted on a total volume of 10 μl, containing 1 μl DNA (4.5 ng μl-1), 5 μl SsoAdvanced™ Universal SYBR® Green Supermix (Bio-Rad Laboratories), 3.4 μl of water and 0.3 μl of each primer (10 μM), using a 96 well plate. The reference genes for the fungus (elongation factor - [9]) and for the plant (elongation factor 1, subunit beta - [33]) are listed in Tab. 1. The following PCR program, which includes the calculation of a melting curve, was used: 95 °C for 30 s, 45 cycles of 95 °C for 10 s, the optimal annealing temperature for 30 s, ramp from 65 to 93 °C with a temperature increment of 0.5 °C and a read plate every 2 s. All the reactions were performed for three biological and three technical replicates. The baseline range and Ct values were automatically calculated using the Bio-Rad CFX Manager software. The fungal reference gene expression was normalized to that of the plant reference gene by subtracting the Ct value of the plant reference gene from the Ct value of the fungal reference gene and obtaining a ΔCt. The measure of the average PCR efficiency was carried out using the software PCR miner ([49]), showing comparable values for the two genes (0.87-0.88). The Kruskal Wallis test was carried out using the free software Past version 3.07 (⇒ http://folk.uio.no/ohammer/past/), with a 0.05 cut-off level of significance.
Gene expression analysis
Gene expression analyses on plant and fungal genes were performed by quantitative real-time (RT-qPCR) on the cDNAs produced starting from the extracted RNAs, using the same machine, the same mix and the same PCR program described above. The primers used are listed in Tab. 1. In order to compare the data from different PCR runs or cDNA samples, the Ct values of all the genes were normalized to the Ct value of the reference. Candidate gene expression was normalized to that of the reference gene by subtracting the Ct value of the reference gene from the Ct value of the candidate gene without efficiency correction, from equation 2-ΔΔCT ([29]), where ΔΔCt represents the ΔCt sample - ΔCt control (control plants). The Kruskal Wallis test was carried out using Past version 3.07, with a 0.05 cut-off level of significance.
Results and discussion
It has been reported that herbivores, such as insects, could have contradictory effects on the mycorrhization ([3], [36]). In this work, the effect of a non-native defoliator on poplar colonization by an AM fungus was compared with that induced by an ecologically related native one, both in total and in partial defoliation. The consequences of defoliation were evaluated at the level of mycorrhizal colonization and on the expression of specific genes, which play a role in symbiosis functioning.
All poplar leaves were defoliated similarly by the two insects, resulting in a 50% defoliation in the partial defoliation experiment and in a 100% defoliation in the total defoliation experiment.
Extent of colonization
AM fungus colonization occurred in both control and defoliated poplars, although it never reached very high levels, as previously reported on the same poplar clone ([27]) and on P. alba ([28]). The colonization was very patchy with some fragments very highly colonized and the most uncolonized. However, morphological observations in the colonized fragments allowed to identify the presence of the typical structures of the symbiosis (such as inter- and intracellular hyphae, coils, vesicles and arbuscules) in the roots of plants, regardless of the thesis (Fig. 1). Here, the low level of colonization, as well as the quality of the root segments after the staining, made morphological quantification difficult, and therefore the presence of the AM fungus has been quantified by calculating the relative fungal DNA abundance (Tab. 2). Quantitative PCR (qPCR) results showed that fungal Cts are higher than plant ones in all the theses, suggesting that fungal DNA is in lower amount than the plant one as previously described ([26]). Statistical analysis showed that there was no significant difference in the amount of fungal DNA in the roots of plants from the different theses (p > 0.05).
Fig. 1 - Cotton blue staining of poplar roots colonized by F. mosseae. Typical AM fungal structures, stained with 0.1% cotton blue, developed in P. nigra “Jean Pourtet” fine roots from F. mosseae-colonized control (a, b) and defoliated plants, with both H. cunea (c, d) and L. dispar (e, f). (a) Intracellular structures (intracellular hyphae and collapsed arbuscules) are visible in a poplar root. Bar = 80 μm. Inset: Detail of a root region where vesicles (V) are visible. Bar = 80 μm. (b) Intracellular arbuscules (A) are present in the root cortex. C, coil. Bar = 50 μm. (c) Root colonization after defoliation with H. cunea. Vesicles (V) along the intercellular hyphae (IH) pervaded the root apparatus. Bar = 50 μm. (d) Arbuscules (A) are visible, as expected, in the root cortex. CC, central cylinder; V, vesicle. Bar= 50 μ m. Inset: Detail of an arbuscule. Bar = 40 μm. (e) Root colonization after defoliation with L. dispar. Inter- and intracellular hyphae and vesicles (V) pervaded the root apparatus. Arrows point on extra-radical mycelium. Bar = 90 μm. (f) A coil (C) is evident among the several inter-and intracellular hyphae (IH). Bar = 60 μm. Inset: Detail of an arbuscule. Bar = 40 μm.
Tab. 2 - Relative DNA quantities of F. mosseae obtained from the qPCR assays on the roots of P. nigra. The Ct value was the mean of three qPCR runs for each DNA sample both for the fungus (F. mosseae) and for the plant (P. nigra). (ΔCt): Ct Gmostef - Ct PopEF1. ΔCts followed by the same letter are not significantly different after the least significant difference test (p > 0.05).
Biological replicate | Ct Gmostef | Ct PopEF1 | ΔCt |
---|---|---|---|
Control plant_C8 | 32.91 | 24.58 | 8.34 a |
Control plant_C9 | 30.20 | 24.31 | 5.89 a |
Control plant_C21 | 30.10 | 23.55 | 6.55 a |
Hyphantria cunea_100_1 | 31.46 | 24.25 | 7.22 a |
Hyphantria cunea_100_2 | 28.01 | 23.94 | 4.07 a |
Hyphantria cunea_100_28 | 28.71 | 24.23 | 4.48 a |
Hyphantria cunea_50_7 | 30.31 | 24.91 | 5.39 a |
Hyphantria cunea_50_12 | 30.36 | 23.86 | 6.50 a |
Hyphantria cunea_50_26 | 36.15 | 23.42 | 12.73 a |
Lymantria dispar _100_5 | 29.33 | 25.21 | 4.13 a |
Lymantria dispar _100_11 | 32.17 | 24.85 | 7.32 a |
Lymantria dispar _100_16 | 31.54 | 25.28 | 6.27 a |
Lymantria dispar _50_4 | 31.63 | 23.83 | 7.80 a |
Lymantria dispar _50_6 | 31.67 | 24.86 | 6.81 a |
Lymantria dispar _50_19 | 29.87 | 25.07 | 4.81 a |
Gene expression analysis
To verify the effect of defoliation on AM symbiosis functioning, the expression of fungal genes, for which a previous role during symbiosis was reported, has been evaluated ([34], [2], [9], [18]). Due to their role in symbiosis, fungal and plant PT genes are considered as key genes for the symbiosis ([2], [7]). Together with the expression of fungal genes, we analyzed a poplar PT gene (PopPT), selected on the basis of the homology with a tomato PT gene (LePT4), which is considered as a marker for a functional symbiosis ([31], [48]).
The ΔCt for each biological replicate was shown in Tab. S1 in Supplementary material, coupled with mean and standard deviation (SD). In Tab. S2 (Supplementary material) the Ct values coupled with mean and standard deviation (SD) were shown for each gene and for each technical replicate.
Starting from the fungal genes, GmosAAP1 gene expression was detected in one-step RT-PCR (data not shown), while it was not possible to quantify its transcripts in RT-qPCR. Cappellazzo et al. ([9]) demonstrated that this gene is expressed in the extra-radical mycelium, but not in the intra-radical one, suggesting a role in the first steps of amino acid achievement and in favoring a direct amino acid uptake from the soil. In poplar-F. mosseae association, it was not possible to quantify its expression by RT-qPCR, while a signal has been detected by one-step RT-PCR. This may suggest that this AM fungus can produce only a small quantity of extra-radical mycelium in our system or that only a little amount of extra-radical mycelium remained attached to the roots after the repotting. Concerning the two H+-ATPases, GmHA5 was expressed in all the five theses and no different expression was assessed in control and defoliated plants (p >0.05 - Fig. 2b), while GmPMA1 transcripts were detected only in one replicate of the defoliated samples using one-step RT-PCR (data not shown), and not in RT-qPCR. Looking at the data previously reported by Requena et al. ([34]), GmHA5 showed a very low expression during non-symbiotic growth and a highly induced expression in mycorrhizal roots and in extra-radical hyphae. GmPMA1 was on the contrary more expressed in the pre-symbiosis and less in the late stages of symbiosis, in agreement with our results. H+-ATPases play a role in the establishment of an electrochemical gradient, necessary for the transport of nutrients across the plasma membrane in both fungi and plants, but each isoform could be engaged at different developmental stages, probably in function of the different necessities in the symbiosis ([34]).
Fig. 2 - Relative transcript level of two F. mosseae and one poplar genes. GmosPT (a: a fungal phosphate transporter, [18]), GmHA5 (b: a fungal H+-ATPase, [34]) and PopPT (c: a poplar phosphate transporter) in presence of a total (100) or partial (50) defoliation carried out by H. cunea (Hc) or L. dispar (Ld). Histograms with the same letter are not significant different after the least significant difference test (p > 0.05).
In this study, no significant differences in GmosPT expression were observed between the control and defoliated samples (p > 0.05 - Fig. 2a), suggesting that the feeding activity of insects has no influence on the phosphate (Pi) transport by the fungus. A role and a control action in Pi transfer by GmosPT was suggested and its expression was reported both in extra- and intra-radical mycelium ([5]). Our results suggest that defoliation does not influence the expression of this fungal gene and that there are no differences between defoliation carried out by the non-native or the native insect considered, at least at the sampling time (eight days after the end of the defoliation). As regards the plant, the expression of the selected poplar PT gene was not affected by the defoliation in any of the theses (Fig. 2c). In tomato mycorrhizal roots LePT4 transcripts have been specifically detected in arbuscule-containing cells together with other tomato PT genes and GmosPT ([2], [18]), suggesting that in arbusculated cells the fungus can compete with the plant for the Pi uptake. Taken together, the results on PT genes, which are considered as markers of a functional symbiosis, confirm the quantitative results, showing no differences in the mycorrhization among the samples.
Additionally, neither the non-native nor the native insect seem to strongly affect the AM colonization, at least at the time of observation.
Previous studies on the impact of defoliation on roots colonized by AM fungi reported controversial results. In Lotus tenuis, the reduction in the photosynthetic capacity by defoliation did not change the root length colonized by AM fungi and the proportion of root colonized by arbuscules, while it decreased vesicular colonization. García & Mendoza ([13]) suggested that the strategy of the AM fungus consisted in investing more of the C resources to preferentially maintain the arbuscules and the inoculum density in the soil by exporting C compounds to retain extra-radical structures such as spores and the hyphal network. In woody species, the extensive below-ground carbon reserves could limit the effects of defoliation on mycorrhizal colonization, even after the loss of large amounts of above-ground biomass ([23]). In particular, the results of a field experiment with the hybrid poplar Populus x canadensis Moench “Eugenei” showed that neither ECM nor AM colonization were affected by L. dispar defoliation during two years. Barto & Rillig ([3]), through a meta-analysis of 99 experiments from 33 publications, suggested that, in contrast with the carbon-limitation hypothesis, herbivory did not reduce mycorrhizal colonization by biologically significant levels in the majority of plants, including perennial grasses and forbs. Recently, Trocha et al. ([42]) have not found changes in the composition of ECM communities of Fagus sylvatica and Pinus sylvestris after damages, including defoliation. On the contrary, Markkola et al. ([30]) and Saravesi et al. ([35]) found changes in the ECM composition when Betula pubescens or P. sylvestris were defoliated, suggesting that high-biomass ECM fungal species need great quantities of carbon because they found a reduction of these fungi after defoliation.
Conclusions
Although the poplar roots showed a low level of AM fungal colonization in all the theses, our results suggest that neither the non-native nor the native insect considered seem to strongly affect the AM colonization, at least in our conditions and sampling time. Two levels of defoliation (total and partial) were considered and the impact of the defoliators on the colonization by an AM fungus was investigated, showing the expression of fungal and plant genes putatively involved in symbiosis functioning. No defoliation theses showed any significant differences compared to the undefoliated ones. Despite several studies simulated herbivory effects on plants using mechanical defoliation or included mechanically defoliated control plants, this approach is deemed appropriate when studying simple biotic interactions, but not necessarily for complex interactions including different trophic levels ([19], [25]), as the ones investigated. Although most of the papers published on AM symbiosis report open field experiments, pot experiments in semi-controlled conditions, like those performed in this work, have allowed to study some of the mechanisms involved in plant-AM fungi interaction under defoliation conditions. However, it cannot be excluded that a longer time after defoliation would be needed to affect fungal colonization. In addition, an analysis of other fungal genes (such as a hexose transporter gene) could be useful to obtain a more complete picture, but unfortunately the F. mosseae genome has not been sequenced and the sequences available are still limited. Interestingly, differential impact of seasonal defoliation on root fungal symbionts has also been reported ([35]). It could be interesting to compare the data obtained in this work employing other defoliators, or to study the AM symbiosis in defoliated poplars directly in nature. As suggested by Saravesi et al. ([35]), different herbivore organisms, which have dissimilar feeding regimes during the growing season, could have diverse effects on the composition or condition of fungal symbionts. Data presented in this study improve the knowledge on differential effect that invasive organisms may have compared to native ones on plant symbionts, an issue recently reported for plant pathogens ([38]), but rarely investigated for defoliators.
Acknowledgements
This work was supported by the Italian Ministry of Education, University and Research, within the FIRB program (grant number RBFR1280NN).
The Authors would like to thank Maria Teresa Della Beffa, Mara Novero and Alessandra Salvioli (Dipartimento di Scienze della Vita e Biologia dei Sistemi, University of Turin, Italy) for the help in mycorrhizal plant preparation and the helpful suggestions on discussion and presentation of AM fungal colonization and molecular data, respectively.
References
CrossRef | Gscholar
CrossRef | Gscholar
Gscholar
CrossRef | Gscholar
CrossRef | Gscholar
Gscholar
CrossRef | Gscholar
Gscholar
Authors’ Info
Authors’ Affiliation
Paolo Gonthier
Dipartimento di Scienze Agrarie, Forestali e Alimentari (DISAFA), Università di Torino, l.go Paolo Braccini 2, 10095 Grugliasco, TO (Italy)
Massimo Faccoli
Dipartimento di Agronomia, Animali, Alimenti, Risorse naturali e Ambiente -Agripolis-, Università di Padova, v.le dell’Università 16, 35020 Legnaro, PD (Italy)
Raffaella Balestrini
Istituto per la Protezione Sostenibile delle Piante, CNR, SS Torino, v.le Mattioli 25, I-10125 Torino (Italy)
CREA - Consiglio per la Ricerca in Agricoltura e l’Analisi dell’Economia Agraria - Unità di Ricerca per le Produzioni Legnose Fuori Foresta (CREA-PLF), str. Frassineto 35, 15033 Casale M.to, AL (Italy)
Corresponding author
Paper Info
Citation
Zampieri E, Petrucco Toffolo E, Mello A, Giorcelli A, Faccoli M, Balestrini R, Gonthier P (2016). Arbuscular mycorrhizal colonization in black poplar roots after defoliation by a non-native and a native insect. iForest 9: 868-874. - doi: 10.3832/ifor1911-009
Academic Editor
Alberto Santini
Paper history
Received: Nov 06, 2015
Accepted: May 21, 2016
First online: Aug 29, 2016
Publication Date: Dec 14, 2016
Publication Time: 3.33 months
Copyright Information
© SISEF - The Italian Society of Silviculture and Forest Ecology 2016
Open Access
This article is distributed under the terms of the Creative Commons Attribution-Non Commercial 4.0 International (https://creativecommons.org/licenses/by-nc/4.0/), which permits unrestricted use, distribution, and reproduction in any medium, provided you give appropriate credit to the original author(s) and the source, provide a link to the Creative Commons license, and indicate if changes were made.
Web Metrics
Breakdown by View Type
Article Usage
Total Article Views: 22143
(from publication date up to now)
Breakdown by View Type
HTML Page Views: 16734
Abstract Page Views: 1051
PDF Downloads: 3070
Citation/Reference Downloads: 63
XML Downloads: 1225
Web Metrics
Days since publication: 2889
Overall contacts: 22143
Avg. contacts per week: 53.65
Article Citations
Article citations are based on data periodically collected from the Clarivate Web of Science web site
(last update: Nov 2020)
Total number of cites (since 2016): 1
Average cites per year: 0.20
Publication Metrics
by Dimensions ©
Articles citing this article
List of the papers citing this article based on CrossRef Cited-by.
Related Contents
iForest Similar Articles
Review Papers
Arbuscular mycorrhizal fungi as a tool to ameliorate the phytoremediation potential of poplar: biochemical and molecular aspects
vol. 7, pp. 333-341 (online: 17 April 2014)
Research Articles
Arbuscular mycorrhizal fungal symbiosis with Sorbus torminalis does not vary with soil nutrients and enzyme activities across different sites
vol. 8, pp. 308-313 (online: 03 September 2014)
Research Articles
Shifts in the arbuscular mycorrhizal fungal community composition of Betula alnoides along young, middle-aged plantation and adjacent natural forest
vol. 13, pp. 447-455 (online: 07 October 2020)
Research Articles
Characterization of two poplar homologs of the GRAS/SCL gene, which encodes a transcription factor putatively associated with salt tolerance
vol. 8, pp. 780-785 (online: 19 May 2015)
Research Articles
Effects of arbuscular mycorrhizal fungi on microbial activity and nutrient release are sensitive to acid deposition during litter decomposition in a subtropical Cinnamomum camphora forest
vol. 16, pp. 314-324 (online: 13 November 2023)
Review Papers
Fifteen years of forest tree biosafety research in Germany
vol. 5, pp. 126-130 (online: 13 June 2012)
Review Papers
Genomics of the Dutch elm disease pathosystem: are we there yet?
vol. 8, pp. 149-157 (online: 07 August 2014)
Short Communications
Detection and quantification of the air inoculum of Caliciopsis pinea in a plantation of Pinus radiata in Italy
vol. 12, pp. 193-198 (online: 10 April 2019)
Research Articles
Early flowering and genetic containment studies in transgenic poplar
vol. 5, pp. 138-146 (online: 13 June 2012)
Research Articles
Effect of plant species on P cycle-related microorganisms associated with litter decomposition and P soil availability: implications for agroforestry management
vol. 9, pp. 294-302 (online: 05 October 2015)
iForest Database Search
Search By Author
Search By Keyword
Google Scholar Search
Citing Articles
Search By Author
Search By Keywords
PubMed Search
Search By Author
Search By Keyword