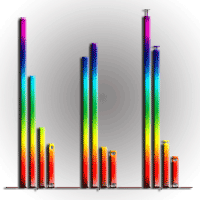
Carbon storage and soil property changes following afforestation in mountain ecosystems of the Western Rhodopes, Bulgaria
iForest - Biogeosciences and Forestry, Volume 9, Issue 4, Pages 626-634 (2016)
doi: https://doi.org/10.3832/ifor1866-008
Published: May 06, 2016 - Copyright © 2016 SISEF
Research Articles
Collection/Special Issue: IUFRO RG7.01.00 - Nice (France 2015)
Global Challenges of Air Pollution and Climate Change to Forests
Guest Editors: Elena Paoletti, Pierre Sicard
Abstract
Land-use changes and afforestation activities are widely recognized as possible measures for mitigating climate change through carbon sequestration. The present study was conducted to evaluate the effect of afforestation on (i) soil physical and chemical properties and soil carbon stocks in four mountain ecosystems and (ii) whole ecosystem carbon storage. The four experimental sites, situated in the Western Rhodope Mountains (Bulgaria) were characterized by typical forest-related land-use conversions. The four sites were a Douglas fir (Pseudotsuga menziesii [Mirb.] Franco) plantation (Rd1) established on former cropland, a mixed black pine (Pinus nigra Arn.) with Scots pine (Pinus sylvestris L.) plantation (Rd2) established on former cropland, a cropland (RdA1) and an abandoned land with uncontrolled extensive grazing (RdA2) historically used as cropland. Soil parameters, i.e., sand content, pH, organic C and N contents, C/N ratio and soil organic carbon (SOC) stocks, were significantly affected by land use and land-use history. Conversion from cropland into forestland significantly reduced soil bulk density and coarse fragments at 0-10 cm depth. Compared with adjacent cropland and abandoned land, soils in coniferous plantations were acidified in their upper layers. Sites Rd2 and RdA2 contained the least SOC owing to the previous long-term arable cultivation (>100 years). Analysis of the ecosystem C stock distribution revealed that most of C in forests was stored in the aboveground tree biomass. Our study confirmed that afforestation of cropland turned the soil into a C sink for the selected mountain region, but showed conflicting results when afforestation occurred on abandoned cropland.
Keywords
Land-use Change, Afforestation, Soil, Forest Floor, Biomass, Carbon Stock
Introduction
Mountain ecosystems are important for their distinct ecological features and the ecosystem services they offer such as regulating atmospheric composition and providing climate change mitigation via carbon (C) sequestration ([42], [64]). Under contemporary global change with widespread abandonment of agricultural lands and massive afforestation activities mostly in the last century, as well as the expected increase in summer temperatures and shifts in precipitation patterns, C storage patterns likely will change in various compartments of mountain ecosystems. Spatial variability of organic matter stocks and turnover in different types of ecosystem depend on many factors (air temperature, aboveground vegetation composition, soil type, soil moisture regime etc. - [27], [37]). Mountain ecosystems are highly sensitive to environmental changes ([32]). A recent report in Europe highlights the fragility and vulnerability of mountain areas ([13]), and projects that both climate and land-use changes (LUC) will have profound effects on their services and functioning. In addition, climate change impacts interact with LUC in mountains ([57], [59]). These changes are expected to affect many services that mountains provide, including C sequestration ([51], [36], [37]).
Environmental degradation, including soil C loss caused by inappropriate land use, is a worldwide problem that has fostered research into sustainable production systems ([75], [19], [62], [29]). Land-use changes influence the balance of soil organic carbon (SOC) and hence may cause CO2 emissions or C sequestration ([47]). In recent years, numerous studies have focused on SOC changes in various ecosystems ([50], [30], [67]). Nevertheless, Poeplau & Don ([47]), showed that in Europe different forms of land use and LUC can lead to either C loss or C accumulation in soils, and emphasized the lack of studies covering all major LUC and biomes with respect to qualitative and quantitative LUC effects.
Soil quality plays an important role in sustainable land management and for environmental integrity. During the recent decades, soil quality concepts have emerged and have been used to assess land or soil quality for various ecosystems ([28], [41]). Changes in land use and land management have great effects on the direction and degree of changes in soil physical and chemical properties ([22]). Land-use change obviously affects the cycles of C ([21], [72]) and nitrogen (N - [49]) but also of soil potassium and phosphorus. Nitrogen is a key factor that influences the primary production of ecosystems ([45]) but, on the other hand, is also controlled by land use and management practices ([69], [68]). With continuous cultivation, physical soil properties may change and organic matter (OM) content, an important carrier of soil quality and storage compound for soil N, may decline ([38]). An important factor for changes in OM content is the flux of plant residues, which is smaller in cropland compared with grassland or forest ([2], [54]). Organic matter input rates also differ among land-use types owing to the different share of root-derived residues ([26], [66]). Many studies have reported significant effects of LUC from cropland to forest and vice versa on soil C ([48], [18]). Conversion of a native ecosystem to cultivated land may cause changes in some soil properties, and often, long-term cropland management induces decreasing soil C stocks. The latter are caused not only by smaller residue inputs but also by less protection of soil C owing to physical disintegration of soil ([9], [5]).
Soil bulk density is the major discriminating factor in soil properties in relation to land use and management ([60], [70], [44]). Soil bulk density integrates effects of management and OM decline; these factors in conjunction result in smaller pore volumes and thus higher bulk densities. Celik ([10]), for instance, showed that soil bulk density increased with cultivation relative to that of adjacent soils in forests and pastures in the southern Mediterranean highlands of Turkey.
In Europe, grasslands at relatively low elevations and flat topography are more and more intensively managed, whereas less fertile pastures at higher altitudes are increasingly abandoned or actively converted into forests ([12]). The area and management of mountain pastures are changing rapidly at national scale ([15]). Despite this rapid change, and in contrast to the vast literature on agricultural soils, only few studies have examined the effects of the long-term conversion from grassland or cropland on soil properties in mountain forest ecosystems ([56]). Historical review on land-use in the mountain zones in Bulgaria showed that past forests have been threatened by excessive and improper use by the local population, which has led to replacement of typical tree species by others ([43], [1]). In Bulgaria, afforestation is of high importance for mountain ecosystems, and substantial land conversion took place during the last decades to contrast erosion, to increase the treeline zone and to use low-productive areas.
Our objective was to characterize soil properties and characteristics of aboveground vegetation in ecosystems of the Western Rhodope Mountains, Bulgaria. We studied the effect of typical regional afforestation practices on soil physical and chemical properties and organic C stocks to evaluate which land-use types are most valuable for ecosystem C storage and, hence, climate change mitigation.
Materials and methods
Study area
The study area was located in the temperate climatic zone and Trace forest region, sub-zone of the Western Rhodopes ([52]). The average annual precipitation in the studied region was 630.5 mm with peaks from May to July, and the mean annual temperature was 7.2 °C. A specific feature of the regional climate is the period of increasing temperatures, which starts from early October to mid-November. The studied sites were located at 860-1270 m a.s.l. in the mountain climatic zone in Bulgaria ([52]).
The study was conducted in 2013/2014 at four experimental sites, representing the main forest-related conversions in this region. The four land-use types included in the study were Rd1, a Douglas fir (Pseudotsuga menziesii [Mirb.] Franco) plantation created on former cropland RdA1; Rd2, a mixed forest plantation dominated by black pine (Pinus nigra Arn.) in association with Scots pine (Pinus sylvestris L.), created on abandoned agricultural land RdA2. At both forest sites, one experimental plot with a total area of 0.1 ha (25 × 40 m) was randomly selected near the adjacent site with maintained previous land use.
Site Rd1 was representative of Douglas fir plantations involving Scots pine and was, together with RdA1, located in the area of St. Petka, Velingrad. The plantation Rd1 was established in 1968 through afforestation in rows on existing cropland. Site RdA1 represented the cropland adjacent to Rd1, and was used for producing annual crops (vegetables and cereals including potatoes, beans, peas, corn, fodder beet, beetroot, rye and oats).
Site Rd2 was part of the area afforested with Scots and black pine plantations before the Second World War in the region of Velingrad for prevention of ongoing soil erosion ([55]). It extended over 2700 ha. The experimental area was located in the locality Baba and Diado on a southern slope. Site Rd2 was the LUC of RdA2. Site RdA2 represented abandoned land, located near Rd2 in the upper part of the south-facing slope. Currently, the area is used occasionally for grazing animals (horses, sheep and goats). Until 80 years ago, RdA2 was managed as a cropland.
Details of the selected sites are given in Tab. 1. Afforestation at both forest sites was performed with 2-year-old seedlings with open root systems and planted with the “Sward of Kolesov”.
Tab. 1 - Characteristics of the experimental sites. (FL): forest land; (CL): cropland; (AL): abandoned land. (*): Age of stand and land-use history (2014); (**): IUSS WG ([25]).
Site | Land-use type | Coordinates | Altitude (m a.s.l.) |
Aspect / slope |
Age / history * | Soil type ** |
---|---|---|---|---|---|---|
Rd1 | Douglas fir plantation | 42.0459498°N 23.9061818°E | 1268 | S /16° | 45 years (CL to FL) |
Dystric Cambisols |
RdA1 | Arable cropland | 42.0456176°N 23.9073822°E | 1246 | S / 25° | 45 years production (potatoes, bean, cereals) (CL remaining CL) | Dystric Cambisols |
Rd2 | Mixed P. nigra and P. sylvestris plantation | 42.0352007°N 23.9698387°E | 860 | S / 11° | 76 years since abandoned cropland (AL to FL) | Dystric Cambisols |
RdA2 | Abandoned land | 42.0364377°N 23.9722885°E | 875 | S / 7° | 80 years ago arable cropland, then abandoned land (CL to AL) | Dystric Cambisols |
Soil sampling and laboratory measurements
Sites were sampled during the growing season between April and October in 2013 and 2014. Soils were sampled randomly in five repetitions within a 0.1 ha experimental plot for all studied land-use types (Tab. 1). All these sites presented similar slope and soil type within the sampled site. Soils were sampled by a hand auger (4 cm in diameter) in four soil layers: 0-10, 10-20, 20-30 and 30-40 cm (20-40 cm depth was analyzed as an aggregated sample of 20-30 and 30-40 cm). In total, 80 soil samples were collected.
Soil properties were determined in accordance with the standardized methods in the Laboratory of Forest Soil Science at the Forest Research Institute - BAS ([11]). Recorded data included morphological field description, bulk density, particle size distribution (wet sieving, including coarse fragments, i.e., particles with a diameter of 2-10 mm), textural classes, pH in H2O ([24]), N content and organic C content. The bulk density was measured per each soil layer of undisturbed soil with cylinders with known volume in two repetitions. Fresh soil samples were individually weighed and dried for 48 h at 105 °C. Each soil sample was sieved in a 2 mm mesh to remove coarse sands while soil aggregates were broken. In the next step, the sample was ground and the total organic C content was measured by the modified method of Tjurin ([3]). Total N content was measured according to the method of Kjeldhal ([7]). The C content, combined with bulk density, was used to estimate the amount of carbon per unit area.
Forest floor (at afforested sites Rd1 and Rd2) and dead litter (RdA1 and RdA2) were sampled within each experimental plot using a 25 × 25 cm frame in three repetitions. Aboveground biomass at RdA1 and RdA2 formed by grasses and crop species was harvested once in the growing season, and biomass was measured by 1 × 1 m frames in three repetitions. All grass species were collected and their fresh weight was measured. Then the grasses were dried in an oven for 48 h at 105 °C and weighed. The samples from forest floor layers and grass species were separated and their dry mass (after 48 h at 105 °C) was measured individually; then each sample was ground. The C content in each layer (L, F and H) was estimated by multiplying the dry weight by the C concentration in dry matter and converted to t C ha-1.
Tree stand characteristics were measured using Bulgarian biometrical methods ([34]). At both forest sites, number of trees, mean diameter at breast height, mean height and growing stock were determined and calculated per hectare. The volumes of biomass were calculated based on tree species tables for trees with bark for the region of the Western Rhodopes ([33], [46]) (Tab. 2), adjusted for stand density. For the present study, we applied IPCC reference values ([23]) for wood dry matter density and C content (1 m3 of wood contains a dry matter of 500 kg with a C fraction of dry matter of 0.5).
Tab. 2 - Biometric characteristics of trees at the studied forest sites (mean ± SE).
Rd | Specie composition | No. trees |
DBH (cm) |
H (m) |
G (m2/0.1 ha) |
V (m3/0.1 ha) |
---|---|---|---|---|---|---|
Rd1 | Pseudotsuga menziesii | 198 | 20.9 ± 0.42 | 22.9 ± 0.23 | 6.792 | 77.25 |
Pinus sylvestris | 5 | 17.9 ± 1.61 | 21.4 ± 1.12 | 0.126 | 1.43 | |
Total/Average | 203 | 20.8 ± 0.41 | 22.8 ± 0.22 | 6.918 | 78.68 | |
Rd2 | Pinus nigra | 146 | 21.3 ± 0.35 | 21.0 ± 0.12 | 5.202 | 56.34 |
Pinus sylvestris | 15 | 24.4 ± 1.28 | 21.7 ± 0.41 | 0.703 | 7.97 | |
Total/Average | 161 | 21.6 ± 0.35 | 21.1 ± 0.11 | 5.904 | 64.31 |
The organic C stocks in soil and litter were quantified according to the IPCC Good Practice Guidance for Land Use, Land Use Change and Forestry ([23]). The SOC stock at each site was based on equivalent soil masses ([14], [65]).
Statistical analysis
Statistics were run using the SPSS 16.0 program. Departure from normal distribution of each soil variable was tested by χ2 normality test. An analysis of variance (ANOVA) was carried out, and differences of means of studied soil properties among depth layers per site and between study sites were verified by Tukey’s test (α = 0.05). Pearson’s linear correlation coefficients among studied parameters were calculated ([58]) and used to reveal the direction of relationships between selected soil properties and among study sites and soil depths.
Results and discussion
Soil bulk density
Bulk density at 0-10 cm depth in the studied land-use types ranged from 0.82 to 0.93 g cm-3. Land use had no significant effect (P = 0.546), although higher densities occurred in cropland (RdA1) and abandoned land (RdA2), and lower densities in the forest stands. In contrast, at 20-40 cm soil depth, bulk densities were slightly higher in the forest stands (0.93 and 0.92 g cm-3) than in abandoned land (0.90 g cm-3 - Tab. 3). Although these values were not significantly different, the relatively higher bulk density in the upper layer of the cropland soil requires additional studies concerning probable indication for soil compaction due to more intensive cultivation by traditional farming and poor crop rotation practices in the region, i.e., a dominance of vegetables and missing cover crops. Some previous studies also indicated that soil cultivation significantly increases soil bulk density ([40]).
Tab. 3 - Main soil and litter/forest floor parameters at the experimental sites (mean ± SE). (DW): Dry weight of forest floor and dead litter.
Site | Layer (thickness, cm) |
Bulk density (g cm-3) |
Sand (%) |
Silt & clay (%) |
Coarse fraction (%) |
DW (g m-2) |
---|---|---|---|---|---|---|
Rd1 | AoL (1.5) | - | - | - | - | 111.93 ± 3.07 |
AoF (1.3) | - | - | - | - | 86.23 ± 3.15 | |
AoH (1.2) | - | - | - | - | 99.03 ± 3.95 | |
0-10 | 0.86 ± 0.04 | 58.9 ± 1.04 | 41.1 ± 1.05 | 21.7 ± 2.24 | - | |
10-20 | 0.88 ± 0.03 | 59.3 ± 1.06 | 40.7 ± 1.07 | 18.5 ± 2.22 | - | |
20-40 | 0.93 ± 0.02 | 62.2 ± 1.10 | 37.8 ± 1.01 | 26.2 ± 2.25 | - | |
RdA1 | AoL (0.2) | - | - | - | - | 14.92 ± 2.45 |
0-10 | 0.93 ± 0.02 | 61.0 ± 1.68 | 39.0 ± 1.73 | 18.9 ± 2.40 | - | |
10-20 | 0.90 ± 0.01 | 66.0 ± 0.62 | 34.0 ± 1.69 | 20.5 ± 2.35 | - | |
20-40 | 0.91 ± 0.04 | 66.0 ± 1.60 | 34.0 ± 1.71 | 26.8 ± 2.37 | - | |
Rd2 | AoL (0.7) | - | - | - | - | 77.48 ± 2.98 |
AoF (1.0) | - | - | - | - | 81.23 ± 3.15 | |
AoH (1.1) | - | - | - | - | 84.32 ± 3.28 | |
0-10 | 0.82 ± 0.03 | 68.9 ± 1.12 | 31.1 ± 1.13 | 13.2 ± 2.39 | - | |
10-20 | 0.88 ± 0.04 | 65.0 ± 1.16 | 35.0 ± 1.09 | 21.2 ± 2.36 | - | |
20-40 | 0.92 ± 0.01 | 67.2 ± 1.11 | 32.8 ± 1.16 | 15.5 ± 2.34 | - | |
RdA2 | AoL (8.0) | - | - | - | - | 178.21 ± 4.19 |
0-10 | 0.92 ± 0.01 | 59.3 ± 1.37 | 40.7 ± 1.26 | 23.0 ± 1.79 | - | |
10-20 | 0.88 ± 0.03 | 60.0 ± 1.2 | 40.0 ± 1.25 | 28.6 ± 1.85 | - | |
20-40 | 0.90 ± 0.03 | 63.4 ± 1.18 | 36.6 ± 1.21 | 28.1 ± 1.72 | - |
Soil coarse fragment distribution
The coarse fragment distribution ranged from 13.2 to 28.6% in different layers of the four land-use types (Tab. 3), but the variability was not related to land use (P = 0.077). The coarse fraction tended to be highest in soil of abandoned land RdA2 (23.0-28.6%), probably because abandonment can result in increased surface stoniness ([31]). This effect could also be related to erosion events and preferential loss of particles <2 mm during previous intensive land management. Under forest vegetation, coarse fragment contents at 0-10 cm depth tended to be low (13.2 and 21.7%). This implies abundant water-stable aggregates that may reduce the soil vulnerability to erosion. For cropland RdA1, the fraction of coarse fragments of between 18.9 and 26.8% indicated that soil in the upper layer was relatively little influenced by erosion but part of the silt fraction may have been lost. Generally, the coarse fragment content in the studied area was low, suggesting good conditions for seedling emergence.
Sand, silt and clay contents
Mean sand content differed significantly between sites (F = 6.050; P = 0.019). Both the highest and the lowest sand contents occurred in the forests (Tab. 3). Considering the three soil depths among sites, sand content was highest at 20-40 cm depth. For all land-use types except Rd2, sand content increased with soil depth. Soil erosion can modify soil properties by reducing soil depth, changing soil texture and inducing loss of nutrients and OM ([16]). Soil silt and clay contents differed significantly between sites (F = 5.943, P = 0.020). This could be due to differences in soil formation but may also be caused by a preferential loss of fine fractions upon erosion ([74]). Sand content increases upon soil erosion after forest conversion and, hence, can be used as an indicator for evaluating soil degradation ([4]). The textural data tentatively suggested that erosion processes at RdA1 were still ongoing. Opposite to sand content, the fraction formed by silt and clay was largest in the superficial soil with highest values for Rd1 and RdA2 (Tab. 3). Plantation Rd2 had the lowest content of medium and small particles (silt and clay). Among sites, sand content was highest in the surface layer of the mixed forest plantation whereas clay content was highest in the surface layer of the RdA2. Unlike the clay fractions in forest sites and abandoned land, that in cropland RdA1 did not change with depth. Based on the data of textural composition, the studied soils were classified as sandy-loams.
Soil pH
Soil pH values were significantly affected by both land use (F = 83.516, P < 0.001) and soil depth (F = 57.693, P < 0.001). Generally, the pH values at the four sites were within the range of moderately acidic to neutral soil reactions as indicated by Foth & Ellis ([17]). Considering the three soil depths, pH was highest in surface layers of RdA1, followed by RdA2 (Fig. 1). By contrast, forest soils were acidified in their upper layers, most likely due to differences in the quality of litter inputs and the acidifying effect of coniferous materials ([6]). Moreover, soil pH at agricultural sites could be increased by management practices. Together, differences in land use came along with systematic and significant differences in soil physical and chemical properties, especially in the superficial soil layer.
Fig. 1 - Soil pH in soil layers. Different letters indicate significant differences among means after Tukey’s test (P ≤ 0.05). Error bars represent the standard error of the mean values per layer for each land use. Land uses compared: (Rd1) Douglas fir forest plantation; (RdA1) Arable cropland; (Rd2) Mixed P. nigra and P. sylvestris forest plantation; (RdA2) Abandoned land.
Carbon content
The average organic C content in mineral soil layers ranged from 0.35 to 4.14% and differed significantly between sites (F = 9.157, P = 0.006). At 0-10 cm depth, the highest C content was found at Rd1 and the lowest at RdA2 (Fig. 2). The highest content in the forest was most likely related to the presence of large biomass and deep-rooting plants that produced root input and prevented easy removal of nutrients from the soil. On the other hand, with conversion from cropland (RdA1) to forest (Rd1), soil C in the 0-10 cm soil layer increased by 3.7% after 40 years. The low C content in cropland RdA1 likely was related to the continuous cultivation of selected crops with high C exports, a factor known to be important for OM decline ([54]). Sites Rd2 (mixed forest land) and RdA2 (abandoned land) had even lower C contents than site RdA1, probably due to previous long-term arable cultivation (>100 years). The forest vegetation at Rd2 caused a small increase in C content at 0-10 cm depth relative to the abandoned land site. An SOC inventory in southeast Germany revealed only slightly lower total SOC stocks in cropland soils compared with forest soils when both top- and subsoils were considered ([67]). In the present study, ANOVA indicated significant differences in C content between the three soil layers (F = 11.046, P < 0.001) for the three land-use types with permanent vegetation, but not for arable cropland (RdA1).
Fig. 2 - Carbon content (%) in soil layers at the study sites. Different letters indicate significant differences among means after Tukey’s test (P ≤ 0.05). Error bars represent the standard error of the mean values per layer for each land use. Land uses compared: (Rd1) Douglas fir forest plantation; (RdA1) Arable cropland; (Rd2) Mixed P. nigra and P. sylvestris forest plantation; (RdA2) Abandoned land.
Nitrogen content
The N content varied between 0.04 and 0.79%, detected at Rd2 (20-40 cm) and Rd1 (0-10 cm), respectively (Fig. 3). Both land use (F = 59.676, P < 0.001) and soil depth (F = 8.225, P = 0.003) were significant factors affecting soil N. The highest N content at 0-10 cm depth was found at Rd1 and the lowest at RdA2. Average N contents at 0-40 cm depth differed between sites Rd1 and RdA1 and between sites Rd2 and RdA2 (P ≤ 0.05 - Tab. 4). It is important to consider that cropland soil generally contains little OM, and thus little organically bound nitrogen, because OM is removed during harvesting. Furthermore, N fertilization of croplands helps to maintain a higher level of soil N as compared with unfertilized fields ([35]), although much of the added nitrogen is exported by harvest. The occasional inputs of fertilizers at study site RdA1 likely did not change the soil total N content. Long-term cultivation and harvesting at this site resulted in smaller SOC and N contents compared with Rd1, despite additional organic fertilization. Moreover, surrounding forest vegetation significantly affected soil N content, with higher values obtained at Douglas fir plantation Rd1 and mixed pine plantation Rd2 than at cropland RdA1 and abandoned land RdA2. This result confirms the findings of Holubík et al. ([20]) at sites in the Czech Republic, where soil development resulted in a higher production of C and N pools in the forest stands compared with agricultural land. Hence, we interpret the different soil N contents largely as being a result of continuous N removal from cropland that was not fully compensated by fertilizer additions.
Fig. 3 - Nitrogen content (%) in soil layers at the study sites. Different letters indicate significant differences among means after Tukey’s test ( P ≤ 0.05). Error bars represent the standard error of the mean values per layer for each land use. Land uses compared: (Rd1) Douglas fir forest plantation; (RdA1) Arable cropland; (Rd2) Mixed P. nigra and P. sylvestris forest plantation; (RdA2) Abandoned land.
Tab. 4 - Carbon and nitrogen contents C/N ratios and C stock in forest floor/dead litter ± standard deviation. Different letters indicate significant differences among the means after Tukey’s test (p ≤ 0.05).
Land-use type | C (%) |
N (%) |
C/N | C stock (t ha-1) |
---|---|---|---|---|
Rd1 | 44.06 ± 4.28 a | 0.63 ± 0.06 b | 70.8 ± 2.9 a | 7.01 ± 0.05 b |
RdA1 | 42.74 ± 5.38 a | 0.83 ± 0.17 ab | 51.7 ± 5.7 b | 0.34 ± 0.01 c |
Rd2 | 44.68 ± 2.88 a | 0.68 ± 0.09 ab | 65.9 ± 4.0 a | 5.78 ± 0.01 b |
RdA2 | 43.25 ± 4.62 a | 0.94 ± 0.08 a | 46.1 ± 1.5 b | 4.11 ± 0.04 a |
C/N ratio
Soil C/N ratios ranged from 3.4 (RdA2) to 15.2 (Rd2), detected at 20-40 cm depth. The difference between the average values along the soil profile at the four sites was statistically significant (F = 9.951, P < 0.001). In the deepest layer, forest soils tended to have higher C/N ratios than cropland and abandoned land (Fig. 4), probably owing to a larger root-derived input.
Fig. 4 - The C/N ratio in soil layers at the study sites. Different letters indicate significant differences among means after Tukey’s test (P ≤ 0.05). Error bars represent the standard error of the mean values per layer for each land use. Land uses compared: (Rd1) Douglas fir forest plantation; (RdA1) Arable cropland; (Rd2) Mixed P. nigra and P. sylvestris forest plantation; (RdA2) Abandoned land.
SOC stock
Cumulated SOC stock differed significantly among sites (F = 44.069, P < 0.001) and soil depths (F = 45.168, P < 0.001). The SOC stock ranged from 8.9 to 35.6 t ha-1 at 0-10 cm depth. It was highest at Rd1 and lowest at RdA2 (Fig. 5). The SOC stock had a C content distribution along the profiles with the highest values at 0-10 cm depth and a general decline with depth (0-10 > 10-20 > 20-30 > 30-40 cm) for all sites but RdA1. The total SOC stock over the entire 0-40 cm soil profile differed by a factor of up to five, ranging from 21 to 103 t ha-1. Abandoned land RdA2 stored the least and forest lands the most carbon in mineral soil. The lowest SOC stock in RdA2 was caused by the low C content but not by a different soil bulk density (Tab. 3). Similarly, Li et al. ([40]) reported that conversion of natural forests to continuous cultivation and grazing decreased both the concentration and stock of SOC. Zhang et al. ([73]) found that SOC stock increased when arable cropland was abandoned. In our case, the lowest SOC stock was found under abandonment (RdA2). In comparison, SOC stock in topsoil was higher at Rd2. If we assume a similar starting condition prior to LUC, there are two possible explanations for this finding. Either there was a smaller SOC accumulation under abandonment, after cropland conversion, as compared with afforestation, or the abandoned site actually lost carbon since conversion. In any case, our results challenge the hypothesis that LUC from cropland to abandoned land might be beneficial for C accumulation in soil.
Fig. 5 - The SOC stock (t ha-1) in mineral soil layers at the study sites. Different letters indicate significant differences among means after Tukey’s test (P ≤ 0.05). Error bars represent the standard error of the mean values per layer for each land use. Land uses compared: (Rd1) Douglas fir forest plantation; (RdA1) Arable cropland; (Rd2) Mixed P. nigra and P. sylvestris forest plantation; (RdA2) Abandoned land.
Our data also indicate that SOC stock increased with elevation, which is consistent with the results of Wang et al. ([63]) and Zhang & McGrath ([71]). However, because of the different starting conditions prior to LUC, we cannot say whether this increase was related to climate and associated gradients in OM decomposition ([61], [39]).
The C content, SOC stock and clay content of all samples correlated highly significantly and positively with land-use type (Tab. 5). Soil C correlated significantly and positively with both N content (r = 0.815, P < 0.01) and clay content (r = 0.500, P < 0.05). The N content was significantly and positively correlated with pH (r = 0.538, P < 0.05) and C content (r = 0.815, P < 0.01) and negatively with sand content (r = -0.518, P < 0.05). These results are in good agreement with findings of Kilic et al. ([30]), who indicated significant correlations between some physical and chemical soil properties under different land uses in Turkey. Our results showed a mutual dependency of OM content and soil physico-chemical properties for soils of the Western Rhodopes and confirmed the role of OM as an ecosystem property ([53]). Our results further showed that total SOC stocks were greater in the forests compared with other sites, and similar findings were reported by Kammer et al. ([27]).
Tab. 5 - Pearson’s correlation matrix for various soil physico-chemical parameters. (**): p<0.01 (2-tailed); (*): p<0.05 (2-tailed).
Parameter | Land use | C content | N content | pH | Sand | Silt & clay |
---|---|---|---|---|---|---|
Land use | 1 | - | - | - | - | - |
C content | 0.824** | 1 | - | - | - | - |
N content | -0.440 | 0.815** | 1 | - | - | - |
pH | -0.182 | 0.347 | 0.538* | 1 | - | - |
Sand | 0.112 | -0.343 | -0.518* | -0.268 | 1 | - |
Silt & clay | 0.654** | 0.500* | 0.218 | -0.180 | -0.398 | 1 |
Forest floor/dead litter
The forest floor formed in both forests was classified as “Moder” type ([8]). The pH was acidic with variations between 4.8 and 4.9 and, according to Tukey’s test, did not differ between both coniferous plantations (Tab. 2). The pH of litter materials from the other land-use types differed significantly among each other and from the forest lands (P < 0.05). The dead litter pH value was highest at RdA2 (abandoned land), where pH was near to neutral in the turf layer.
The dry weight of forest floor/dead litter differed significantly (P < 0.05) among the four sites. It was highest under abandonment (RdA2: 178.21 g m-2) and lowest under cropland use (RdA1: 14.92 g m-2). Sites RdA1 and RdA2 had completely different depth profiles, and the formed turf layer in the abandoned grassland likely played an important role in formation of plant inputs towards the mineral soil. On the other hand, the regular harvest export in the cropland resulted in very small amounts of litter.
The C content in forest floor/dead litter was not significantly different among the four sites (between 42.7 and 44.7% - Tab. 4). The N content varied significantly between land-use types (P < 0.05). It was lowest in the coniferous plantations (0.63-0.68%) and highest under cropland and abandoned land use (0.83 and 0.94%, respectively). The differences in C/N ratios between sites were statistically significant (P < 0.05, range 46.1-70.8) and indicated higher litter qualities, i.e., better decomposability for litter in non-forests than in forests. The C/N ratios were highest in forest floor litter (Rd1 > Rd2) and lowest in abandoned land litter (RdA2).
Forest floor/dead litter C stocks differed significantly between the four sites (F = 69.132, P < 0.001). Overall mean values for forest plantations were 5.78 and 7.01 t ha-1 for Rd2 and Rd1, respectively (Tab. 4). The cropland had the lowest dead litter C stock (0.34 t ha-1). A high dead litter accumulation on the abandoned land suggested a reduced microbial activity at this site or higher input of dead organic materials. Statistically significant and positive correlation was found for pH and N content (r = 0.748, P < 0.01), whereas the C/N ratio correlated significantly negatively with pH (r = -0.910, P < 0.01) and indicated that processes of nitrification of organic materials were related with pH.
Aboveground vegetation
The C stock was higher in the aboveground biomass of forests than of cropland or abandoned land (Fig. 6). Site Rd1 stored the most carbon in vegetation (196.7 t ha-1), followed by Rd2 (160.8 t ha-1). A substantial amount of carbon was accumulated also in aboveground parts of abandoned land, but more studies are required in order to reveal the seasonal variation and to estimate the annual mean. Concerning the aboveground tree biomass and carbon stored in it, more detailed studies are required to obtain data for branches and needles. The latter two are not explicitly considered in the growing tables applied in national forestry, especially for Douglas fir stands.
Fig. 6 - Carbon stock distribution in ecosystem compartments of studied land-use types. Different letters indicate significant differences among means after Tukey’s test (P ≤ 0.05). Error bars represent the standard error of the mean per layer for each land use. Land uses compared: (Rd1) Douglas fir forest plantation; (RdA1) Arable cropland; (Rd2) Mixed P. nigra and P. sylvestris forest plantation; (RdA2) Abandoned land. Compartments included are: Soil, FF/litter - forest floor (F+H) and litter layer, Aboveground - tree and grass vegetation.
Carbon stock distribution in ecosystem compartments
The C stock distribution in different ecosystem compartments showed that in forest land and under abandonment, aboveground vegetation represented the largest C pool. The amount of carbon in aboveground vegetation was similar for both forest stands, although they consisted of different coniferous species and with different stand characteristics (Fig. 6). The forest floor was another important C pool at the forest sites. At the abandoned land site, both dead litter and standing biomass contributed the most to the overall ecosystem C storage whereas mineral soil C played a minor role. At the arable cropland site, carbon was mostly stored in the mineral soil whereas fresh litter was removed from the system via harvest, leaving little litter material in the ecosystem.
Conclusions
Land-use change in the Western Rhodope Mountains is one of the major drivers for modulating soil characteristics. We found significant changes and variations of soil C storage down to 40 cm of soil depth, although differences were most pronounced in the superficial 0-10 cm of organo-mineral soil. Afforestation with coniferous tree species caused changes in soil pH expressed as acidification of the superficial soil layers. Typical mountainous agricultural land use was related with changes in textural fractions suggesting ongoing processes of superficial erosion. Different soil N contents were most significant between Rd1 and RdA1 as a result of continuous N removal from arable cropland (RdA1) that was not fully compensated by fertilizer additions. It could be summarized that due to afforestation activities, the N content in upper soil layers increased.
Afforestation helped accumulate and preserve soil C, but even several decades after the conversion from arable cropland or abandoned land to forest land use, this effect was expressed only weakly and could not compensate the organic C loss due to previous active cultivation. The C content in soil and its profile distribution were shown to be very sensitive to land-use and coverage changes and could be proposed as appropriate indicators for studying the long-term effects of LUC on soil properties. Of all studied compartments of mountain ecosystems, mineral soil remained the main accumulator of SOC for all studied land-use types. Together with mineral soil, aboveground tree vegetation also accumulated a major part of carbon at the studied forest and abandoned sites.
Afforestation considerably increased organic C stock in the uppermost soil layers of different land-use types in the Western Rhodope Mountains. Afforestation could be identified as a good practice that could preserve soil C and improve the regulating and sustaining ecosystem services of mountain regions. Therefore, we recommend conserving mountain soils by preventing abandonment and by establishing appropriate forest plantations.
Acknowledgments
The work was a part of the project “Land-use and management impacts on carbon sequestration in mountain ecosystems” financed within the framework of the Bulgarian-Swiss Research Programme (BSRP), 2011-2016, between the Government of Bulgaria and the Swiss Federal Council, contract number IZEBZ0_142972.
References
Gscholar
Gscholar
Gscholar
Gscholar
Gscholar
Online | Gscholar
Gscholar
Gscholar
Gscholar
Gscholar
Gscholar
Gscholar
Gscholar
Gscholar
CrossRef | Gscholar
Gscholar
Gscholar
CrossRef | Gscholar
Gscholar
Authors’ Info
Authors’ Affiliation
Forest Ecology Department, Forest Research Institute, BAS, “Kl. Ohridski” Blv., 1756 Sofia (Bulgaria)
Department of Forest Genetics, Physiology and Plantations, Forest Research Institute, BAS, “Kl. Ohridski” Blv., 1756 Sofia (Bulgaria)
Forestry Department, Forest Research Institute, BAS (Bulgaria)
Corresponding author
Paper Info
Citation
Zhiyanski M, Glushkova M, Ferezliev A, Menichetti L, Leifeld J (2016). Carbon storage and soil property changes following afforestation in mountain ecosystems of the Western Rhodopes, Bulgaria. iForest 9: 626-634. - doi: 10.3832/ifor1866-008
Academic Editor
Elena Paoletti
Paper history
Received: Sep 08, 2015
Accepted: Dec 30, 2015
First online: May 06, 2016
Publication Date: Aug 09, 2016
Publication Time: 4.27 months
Copyright Information
© SISEF - The Italian Society of Silviculture and Forest Ecology 2016
Open Access
This article is distributed under the terms of the Creative Commons Attribution-Non Commercial 4.0 International (https://creativecommons.org/licenses/by-nc/4.0/), which permits unrestricted use, distribution, and reproduction in any medium, provided you give appropriate credit to the original author(s) and the source, provide a link to the Creative Commons license, and indicate if changes were made.
Web Metrics
Breakdown by View Type
Article Usage
Total Article Views: 22378
(from publication date up to now)
Breakdown by View Type
HTML Page Views: 16476
Abstract Page Views: 1179
PDF Downloads: 3604
Citation/Reference Downloads: 28
XML Downloads: 1091
Web Metrics
Days since publication: 3004
Overall contacts: 22378
Avg. contacts per week: 52.15
Article Citations
Article citations are based on data periodically collected from the Clarivate Web of Science web site
(last update: Nov 2020)
Total number of cites (since 2016): 8
Average cites per year: 1.60
Publication Metrics
by Dimensions ©
Articles citing this article
List of the papers citing this article based on CrossRef Cited-by.
Related Contents
iForest Similar Articles
Short Communications
Variation in soil carbon stock and nutrient content in sand dunes after afforestation by Prosopis juliflora in the Khuzestan province (Iran)
vol. 10, pp. 585-589 (online: 08 May 2017)
Research Articles
Effects of tree species, stand age and land-use change on soil carbon and nitrogen stock rates in northwestern Turkey
vol. 9, pp. 165-170 (online: 18 June 2015)
Research Articles
Soil stoichiometry modulates effects of shrub encroachment on soil carbon concentration and stock in a subalpine grassland
vol. 13, pp. 65-72 (online: 07 February 2020)
Research Articles
Effects on soil characteristics by different management regimes with root sucker generated hybrid aspen (Populus tremula L. × P. tremuloides Michx.) on abandoned agricultural land
vol. 11, pp. 619-627 (online: 04 October 2018)
Research Articles
Thinning effects on soil and microbial respiration in a coppice-originated Carpinus betulus L. stand in Turkey
vol. 9, pp. 783-790 (online: 29 May 2016)
Research Articles
Estimating changes in soil organic carbon storage due to land use changes using a modified calculation method
vol. 8, pp. 45-52 (online: 17 June 2014)
Research Articles
Estimation of fuel loads and carbon stocks of forest floor in endemic Dalmatian black pine forests
vol. 13, pp. 382-388 (online: 01 September 2020)
Research Articles
Carbon stock in Kolli forests, Eastern Ghats (India) with emphasis on aboveground biomass, litter, woody debris and soils
vol. 4, pp. 61-65 (online: 05 April 2011)
Research Articles
Changes in the properties of grassland soils as a result of afforestation
vol. 11, pp. 600-608 (online: 25 September 2018)
Review Papers
Soil fungal communities across land use types
vol. 13, pp. 548-558 (online: 23 November 2020)
iForest Database Search
Search By Author
Search By Keyword
Google Scholar Search
Citing Articles
Search By Author
Search By Keywords
PubMed Search
Search By Author
Search By Keyword