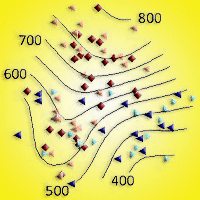
Changes in vegetation diversity and composition following livestock removal along an upland elevational gradient
iForest - Biogeosciences and Forestry, Volume 8, Issue 5, Pages 582-589 (2015)
doi: https://doi.org/10.3832/ifor1557-008
Published: Apr 22, 2015 - Copyright © 2015 SISEF
Research Articles
Abstract
European heathland habitats are cultural landscapes derived from previously-forested ecosystems. Heathlands are of significant conservation interest but have experienced prolonged degradation due to a range of factors including overgrazing by domestic livestock. There is growing recognition of the need to restore upland landscapes to produce a diverse mosaic of woodlands, heathlands and forest edge ecotones. In some studies stock removal has been sufficient to promote heathland recovery, but often more intensive interventions are required. Few studies have specifically examined how abiotic gradients associated with changing elevation might relate to restoration success. We examined differences in vegetation between grazed and restored areas over a 500 m elevational gradient split across two hillsides that were part of a landscape-scale restoration project in the Scottish Southern Uplands. Species alpha and gamma diversity showed non-linear responses to elevation but the effects of grazing differed between sites. Grazing increased diversity on the lower elevation site but reduced it at higher elevations. The differing effects of grazing with elevation can be interpreted in the context of levels of competition and likely impacts on rates of colonization and extinction. Differences in community composition were assessed using PERMANOVA, NMDS and Cluster Analysis and were primarily controlled by elevation with no significant effect of grazing. The keystone heathland species Calluna vulgaris was not recorded in any of our monitoring plots but some other dwarf shrubs were common. Changes in community structure following stock removal are slow on upland sites but initial impacts interact strongly with abiotic site conditions and pre-restoration vegetation composition. During large-scale restoration it is therefore vital to consider how widely-applied treatments might differ in their effects across landscapes. Changes in diversity may provide a useful early indicator of important ecological processes and likely directions of change.
Keywords
Calluna vulgaris, Community Change, Grazing, Heathland, Moorland, Restoration, Scotland
Introduction
Calluna vulgaris-dominated heathlands are cultural landscapes, derived from previously-forested ecosystems, which have been subject to human manipulation and management for several millennia. Heathlands are of international conservation importance ([56], [24]) and this is recognized in national and international legislation ([39], EU Habitats Directive 92/43/EEC). They owe their open character to low-intensity traditional livestock grazing and managed burning ([60], [20]). Habitat degradation, associated with poorly-managed livestock grazing is, however, a global issue facing many tropical, semi-arid and temperate ecosystems ([42], [18], [19]). Significant potential exists for livestock grazing to induce dramatic changes in ecosystem state from which restoration can be difficult ([55]). Throughout Europe large areas of heathland habitat have become increasingly degraded over the last century ([19]). Calluna-dominated communities have been lost due to changes to managed fire regimes ([6]); nutrient deposition associated with atmospheric pollution ([23], [9]); acid deposition ([7]); and land-use conversion ([28]). In the United Kingdom there are a growing number of agencies and organisations focused on landscape-scale ecosystem restoration in the uplands. Objectives are often focused on tree planting and the restoration of forest ecosystems (see [29] for an overview), but there is also recognition of the desirability of restoring forest ground flora and a diverse mosaic of habitat types that includes woodlands, heathlands and forest edge ecotone communities ([32]). Degraded heathlands are often dominated by competitive grass species such as Molinia caerulea (purple moor grass) and Nardus stricta (moor matgrass). Both of these can attain high levels of abundance, lead to communities with relatively low levels of diversity and can pose significant challenges for restoration practice ([25], [40]). A number of studies have examined the effects of grazing regimes on heathland communities, often concluding that restoration prescriptions need to be site-specific. For example, whilst it has proved possible to facilitate heathland recovery by simply reducing grazing pressure ([3], [30], [31]), often it has taken additional interventions to allow Calluna establishment. Successful treatments have included soil disturbance by cattle trampling, rotavation, herbicide application and reseeding ([40], [41]) and, where nutrient enrichment is an issue, topsoil removal ([58]). Such intensive restoration actions may require managers to make difficult trade-offs as they may maximize Calluna cover but can also lead to the loss of other components of the community ([36]).
Many of the differences seen between studies can be ascribed to variation in the initial floristic composition of the sites undergoing restoration, and the abundance of Calluna within the community or seed-bank. A few studies have taken a multi-site perspective and assessed interactions between restoration and site edaphic characteristics and initial community composition ([50], [61], [13], [40]). These have allowed authors to examine the extent to which general patterns can be discerned in post-restoration vegetation development, but untangling the effects of site-to-site differences in management history, vegetation, climate and soil type can still be difficult. Körner ([35]) points to the power of elevational gradients in testing the response of systems to geophysical influences. It is long-established that edaphic characteristics play a strong role in defining the landscape-scale distribution of upland vegetation communities ([59]). However, variation in edaphic characteristics, including differences in soil type, soil fertility, climate and productivity, across abiotic ecotones can also interact with management to drive changes in vegetation communities ([15], [47], [57]).
This research took advantage of a real-world, landscape-scale restoration treatment to determine how initial vegetation responses to grazing removal interacted with the wide variation in elevation across the site. Approximately half of our study-site was fenced-off in a single, landscape-scale exclosure during autumn 2010 (three years prior to this study). The site managers’ aim was to allow establishment of planted native woodland and the recovery of semi-natural vegetation at the woodland edge and in sub-montane and montane zones. Since elevational gradients are known to be associated with patterns in both species diversity and overall community composition, we were interested in assessing whether there was variation in restoration potential with elevation and if elevation influenced the resistance of communities to change. Our specific objectives therefore included: (1) assessing how grazing removal and elevation interact to drive changes in species diversity; and (2) quantifying the extent to which grazing and elevation determine community composition.
Material and methods
Study site
Research was completed at Corehead, a 640 ha hill farm near Moffat in the Southern Uplands of Scotland (lat: 55° 23′ 42″ N, long: 3° 27′ 54″ W). Elevation at Corehead ranges from 200 to 800 m a.s.l. Local rainfall averages 1635 mm year-1 with average annual maximum and minimum temperatures of 11.0 and 3.6 °C, respectively ([1]). Corehead has been the property of the Borders Forest Trust since 2009, and they aim to maintain low intensity upland sheep farming on a proportion of the site whilst restoring a mosaic of native habitats, including deciduous woodland, heathland and montane scrub.
Research was split between two study sites - Linshaw Knowe (max. elevation 500 m) and Hartfell (max. elevation 808 m). Soil types (as per [52]; WRB soil types provided in brackets) ranged from brown earths (cambisols) at the lowest elevations, through peaty podzols (umbrisols/podzols) at higher elevations to montane soils (umbrisols) on the summit of Hartfell. Underlying geology consists of greywackes (sandstone) with shale bands. Prior to restoration, vegetation at Corehead was typical of the relatively heavily grazed hills of northern and western Great Britain. At Linshaw Knowe it was dominated by a species-poor mixture of Festuca ovina - Agrostis capillaris - Galium saxatile grassland (National Vegetation Classification, NVC - [51], community U4); Nardus stricta - Galium saxatile grassland (NVC U5) and Pteridium aquilinum - Galium saxatile - Anthoxanthum odoratum grassland (NVC U20b) ([1]). On Hartfell vegetation graded from Nardus stricta - Galium saxatile grassland (NVC U5) into Carex bigelowii - Racomitrium lanuginosum moss-heath (NVC U10) at the highest elevations ([1]). Calluna was absent from large areas of the study site but patches remained in areas naturally-protected from grazing (steep slopes, gullies, etc.). The presence of such patches, combined with local oral history, suggests these habitats were formerly more extensive.
Stock were removed from half of Corehead in 2010 using a landscape-scale exclosure. Prior to removal stock numbers varied across the site. There were approximately 2.8 ewes ha-1 on Linshaw Knowe and 1.6 ewes ha-1 on Hartfell. A small number of cattle were also grazed during the summer. Historic grazing pressure is likely to have been substantially higher. Nutrient export from livestock production can compensate for atmospheric inputs ([21]) and thus, on managed heathlands like our study-site, loss of Calluna dominance is mostly associated with over-grazing by livestock ([54], [2]).
Design and monitoring
Pseudoreplication (sensu [33]) is a troublesome though unavoidable issue in “natural experiments” such as ours. Pseudoreplication can arise when researchers define a hypothesis and collect what they deem to be independent samples from two populations they wish to compare. In reality the samples are not independent because, for example, each sample is collected from a single monitoring unit and treatment effects can be confounded with background variation in biotic and abiotic conditions. We agree with Hargrove & Pickering ([27]), Oksanen ([44]) and Kozlov & Hurlbert ([34]) that this means hypotheses need to be framed carefully, acknowledging what statistical inferences can and cannot be made. As our site consists of a single exclosure we acknowledge at the outset that samples taken in grazed and ungrazed areas can only be considered independent samples of each side of the fence at our specific site. We therefore test the hypothesis that there are differences in vegetation diversity and composition on either side of the fence at our site. We cannot conclude on the basis of our statistical analysis that any differences observed are the result of grazing removal. As Hargrove & Pickering ([27]) point out, we can form “how possibly” hypotheses to suggest explanations for the patterns we observe.
Study sites were selected to allow direct comparisons of communities still used for sheep grazing and those that had been protected by fencing for nearly three years. We aimed to capture variation in the effects of grazing across an ecotone that included a transition from the moorland-fringe into montane communities. Due to the position of fencing and land-ownership this involved splitting the ecotone across the two sites previously described. Monitoring plots were established at 50 m intervals in elevation including three elevations on Linshaw Knowe (400-500 m) and five on Hartfell (600-800 m). Locations were chosen to have similar aspects (S-SSW), though the convex profile of the hillslopes meant slopes declined with elevation at both sites. At each elevation we placed ten 1 × 1 m monitoring plots (five on each side of the fence) along the contour at 10 m intervals from the boundary. The two groups of plots, grazed and ungrazed, at each elevation were deliberately located close to each other and to the fence, to ensure that management history and underlying hydrological and soil conditions were similar.
Within each plot we made visual estimates of the cover (nearest 1 %) of all vascular plant species and litter cover. Cryptogams were recorded by functional group (acrocarpous mosses, lichens pleurocarpous mosses, Sphagnum spp.). A number of species could not be readily distinguished in the field. In particular it proved difficult to reliably separate Carex bigelowii (Bigelow’s sedge) from montane forms of Carex nigra (common sedge), whilst Agrostis canina (velvet bent) and Agrostis vinealis (brown bent) could not be distinguished without causing undue disturbance to the plots. These species were therefore pooled for analysis. Species nomenclature follows Stace ([53]). The raw data used in the analysis are provided in Appendix 1.
Data analysis
Species richness and diversity
Species richness and species diversity (Shannon-Weiner Index) were calculated for all eighty plots using the “specnumber” and “diversity” functions in the “vegan” package ([45]) of the software R version 2.15.0 ([49]). Differences in richness and diversity were analyzed using a linear model in R. We tested for the effects of site, SoF (side of the fence - grazed or ungrazed) and elevation. Since site and elevation were confounded, we specified elevation as nested within sites. We analyzed changes in litter cover (square root transformed) in the same way.
Differences in beta diversity across elevations and between sides of the fence were characterized using the “betadisper” function in the “vegan” package of R with the Bray-Curtis Dissimilarity Index based on all species and raw abundances ([5]). Beta diversity was defined as the average distance to the group centroid for each combination of grazing status and elevation. We used a linear model to test for differences in beta diversity according to SoF and elevation within sites. We also estimated gamma diversity for each elevation/ grazing combination by calculating the total number of species recorded across the relevant subset of plots.
Community composition
We used hierarchical agglomerative cluster analysis to identify recognizable community types across our site. Analysis was completed with the “hclust” function in R using the Bray Curtis dissimilarity index and Ward’s method. The appropriate number of groups was identified by examining scree plots while considering the need for ease of interpretation.
We tested for the effects of site, SoF and elevation on species composition using Permutational Multivariate Analysis of Variance (PERMANOVA - [4]). Tests were completed in R using the “adonis” function in the “vegan” package. Here we were interested in broad differences in community composition so we removed rare species (those occurring in < 5% of plots) as these can exert a strong influence on differences between plots. Prior to analysis we also applied a “wisconsin” double standardization (by species maximum and total plot cover). Analysis was completed using the Bray-Curtis Dissimilarity Index and 10 000 permutations. As site was confounded with elevation we first tested for the effects of site and SoF (including interactions) before examining the effects of elevation and SoF. For the latter test we restricted permutations to within sites.
Non-Metric Multidimensional Scaling (NMDS) was used to visualize variation in plot composition according to differences in site, elevation and grazing status. Analysis was completed using the “metaMDS” function in the “vegan” package of R. Data was pre-treated in the same way as for the PERMANOVA analysis. We examined one to six dimensional solutions and used a scree plot of stress versus number of dimensions to select a solution that provided an adequate balance between simplicity and low stress. We investigated the relationship between plot composition and elevation using the “ordisurf” function. “ordisurf” uses a General Additive Model to fit a smooth surface for an environmental variable and plots the result on an ordination diagram ([8]). We selected the optimal model complexity by varying the number of knots to minimize Generalized Cross Validation prediction error (GCV).
Results
Species richness and diversity
There were significant three-way interactions between site, SoF and elevation for both species richness (F[4, 72] = 4.33; p = 0.003) and species diversity (F[4, 72] = 5.03; p = 0.001). Differences in species richness and diversity on each side of the fence are conditional on site-specific, differing responses to changing elevation. Given the difficulty of interpreting such complex interactions we fitted two additional models to investigate the within-site effects of SoF and elevation (Tab. 1).
Tab. 1 - Results of linear models examining within-site effects of side of the fence (SoF ~ grazing status), elevation and their interaction on species richness (top) and species diversity (Shannon-Weiner Index; bottom). For all tests the d.f. for each term were 1.26 for Linshaw Knowe and 1.46 for Hartfell.
Group | Parameter | Linshaw Knowe | Hartfell | ||||
---|---|---|---|---|---|---|---|
Coeff. | F | p | Coeff. | F | p | ||
Richness | SoF | -17.167 | 7.71 | 0.010 | 9.560 | 0.68 | 0.415 |
Elevation | -0.016 | 0.02 | 0.904 | -0.002 | 8.89 | 0.004 | |
SoF × Elevation | 0.034 | 4.26 | 0.049 | -0.013 | 5.75 | 0.021 | |
Diversity | SoF | -2.850 | 1.60 | 0.217 | 1.912 | 0.82 | 0.370 |
Elevation | -0.005 | 1.68 | 0.206 | -0.001 | 10.07 | 0.003 | |
SoF × Elevation | 0.006 | 3.84 | 0.061 | -0.002 | 5.28 | 0.026 |
For species richness, there were significant interactions between grazing and elevation at both sites. On Linshaw Knowe richness increased with elevation in ungrazed plots but declined in grazed plots (Fig. 1). On Hartfell species richness decreased with elevation on both grazed and ungrazed sides but the effect was noticeably stronger for those that were ungrazed.
Fig. 1 - Average plot species richness as a function of site and elevation. Linshaw Knowe is shown as triangles and Hartfell as squares. Grazed and ungrazed plots are shown as dark and light colors, respectively. Error bars are ± one standard deviation.
Species diversity was controlled by the interacting effects of SoF and elevation on Hartfell (Fig. 2). Diversity declined with elevation for both grazed and ungrazed plots but the effect was stronger for ungrazed plots. On Linshaw Knowe there was no significant effect of SoF or elevation on diversity. However, the interaction between these two effects was only marginally non-significant (Tab. 1). No significant effects of SoF or elevation were detected on beta diversity.
Fig. 2 - Average plot species diversity (Shannon-Weiner Index) as a function of site and elevation. Linshaw Knowe is shown as triangles and Hartfell as squares. Grazed and ungrazed plots are shown as dark and light colors, respectively. Error bars are ± one standard deviation.
Differences in gamma diversity were a result of both grazing status and elevation (Fig. 3). Gamma diversity declined monotonically for grazed plots but appeared to follow a unimodal distribution on ungrazed plots. Gamma diversity was, in general, higher for grazed plots on the low elevation site but the opposite was true on the higher elevation site.
Fig. 3 - Total species richness (gamma diversity) across all plots in a given elevation/grazing combination. Linshaw Knowe is shown as triangles and Hartfell as squares. Grazed and ungrazed plots are shown as dark and light colors, respectively.
There was a significant three-way interaction of site, SoF and elevation on litter cover (F[4, 72] = 4.19; p = 0.004). Site-level models revealed significantly higher cover in ungrazed plots on Linshaw Knowe (F[1, 26] = 38.63; p < 0.001) and a significant decline in cover with elevation on Hartfell (F[1, 46] = 11.65; p = 0.001).
Community composition
Cluster analysis identified six community groups that accounted for 76% of the variation in the dataset (Tab. 2, Fig. 4). No groups consisted solely of grazed or ungrazed plots. Community composition was best visualized by an NMDS with four dimensions (stress = 0.15). The effect of elevation could be fitted to the resulting ordination using an ordisurface with 15 knots (GCV = 6822, R2adj = 0.71). The results showed that Linshaw Knowe and Hartfell host a number of distinct community types (Fig. 3), but there was a substantial degree of overlap at mid-elevations, where communities were dominated by Agrostis spp., N. stricta, Deschampsia flexuosa (wavy hair grass) and Vaccinium myrtillus (blaeberry - Fig. 4). The latter species was the only dwarf-shrub recorded and, on Hartfell, was dominant enough in places to form a separate community-type. M. caerulea was common across both sites, all elevations and both grazing treatments, but was particularly associated with the lower and upper elevations of Linshaw Knowe and mid-elevations on Hartfell. The highest elevations on Hartfell were clearly distinguished by the abundance of C. bigelowii/C. nigra and Juncus squarrosus (heath rush - Fig. 4). Whilst sites and elevations could be clearly distinguished in the ordination there was no obvious effect of SoF. This was confirmed by the results of the PERMANOVA: the first test revealed a significant effect of site (pseudo-F = 8.4, p < 0.001) but no effect of SoF or site/SoF interaction; the second test showed a significant effect of elevation within sites (pseudo-F = 11.8, p < 0.001) but no effect of SoF or grazing/elevation interaction.
Tab. 2 - Community groups identified by cluster analysis. The table gives a community name and lists the dominant species (those with a mean relative cover > 0.1). Frequency shows the number of plot community members with the numbers in brackets showing the number of grazed and ungrazed plots respectively. Symbol refers to the plotting symbol used for the community type in Fig. 4.
Community group | Dominant species | Frequency | Symbol |
---|---|---|---|
Blaeberry heath | Vaccinium myrtillus, Agrostis canina, Potentilla erecta | 7 (4/3) |
Circles |
Mixed grassland | Potentilla erecta, Deschampsia flexuosa, Festuca ovina | 27 (14/13) |
Squares |
Bedstraw-bent grass grassland | Galium saxatile, Agrostis canina, Carex flacca, Molinia caerulea | 6 (4/2) |
Diamonds |
Bigelow’s sedge montane grassland | Carex bigelowii/nigra, Festuca ovina | 20 (10/10) |
Gray triangles |
Heath rush sub-montane grassland | Juncus squarrosus, Carex bigelowii/nigra, Festuca ovina, Molinia caerulea | 6 (3/3) |
White triangles |
Purple moor grass grassland | Molinia caerulea, Scirpus cespitosus, Potentilla erecta | 14 (5/9) |
Stars |
Fig. 4 - NMDS ordination showing: (A) plots in relation to their site and grazing status (triangles: Linshaw Knowe; squares: Hartfell; dark: grazed; light: ungrazed; hulls surround all plots on a site); (B) species centroids (see Appendix 2 for the list of species codes); (C) plots in relation to elevation, site and grazing status; (D) plots in relation to their community groups (see Tab. 1 for symbol codes).
Discussion
Changes in species richness and diversity
Previous research has recorded differing changes in heathland species diversity due to grazing control. Positive effects of grazing on diversity have been recorded by: (i) Bullock & Pakeman ([13]) who showed that grazing on lowland heath increased species richness by reducing shrub and scrub cover at the expense of grasses and forbs; (ii) Bokdam & Gleichman ([10]) showed increase in richness in Calluna and D. flexuosa dominated habitats in response to grazing by cattle; (iii) Britton et al. ([12]) recorded increased richness in Racomitrium heath in response to heavy sheep grazing. In contrast, negative effects on species richness were recorded by Britton et al. ([12]) in montane Vaccinium heaths. Other authors have found limited or mixed effects, for example Britton & Fisher ([11]) found no effect of simulated grazing on the species richness of low-alpine Calluna heathland and Vandvik et al. ([57]) found grazing led to reduced site-level species richness in dry heath but increased richness in moist heath.
Olff & Ritchie ([46]) reviewed the ways in which grazing can affect the diversity of plant communities depending upon the intensity of grazing, grazer body size and site abiotic conditions. They also drew attention to the fact that the effects of grazing will vary across environmental gradients. Our analysis revealed complex relationships between species diversity and site, elevation and the side of the fence on which plots were located. As our study examined a single exclosure we cannot statistically state that differences between sides of the fence are due to grazing per se. However, with no major differences in aspect, soil moisture or soil type between grazed and ungrazed plots within each elevational band, there appears to be few other reasonable explanations for the patterns we observed. For both species richness and diversity there were significant three way interactions between the three variables included in our statistical models (site, elevation and side of fence). This complexity was a result of both differing elevational changes in diversity between grazed and ungrazed plots and an apparent unimodal distribution of richness and diversity across the entire gradient (Fig. 1 and Fig. 2). Gamma diversity displayed a similar pattern for ungrazed plots but there was a monotonic decline for grazed plots (Fig. 3). A number of explanations have been put forward to explain “humpbacked” patterns of diversity in response to elevation. These include the effect of range overlaps in transitions between zonal communities ([38]), the “mass effect” ([26]) and the “mid-domain effect” ([14]). Variations in management history (e.g., grazing intensity) are also likely to be important. The patterns we have recorded in alpha and gamma diversity suggest that changes to grazing regimes can modify processes controlling landscape-scale patterns of biodiversity. The removal of grazing seemed to alter the patterns of alpha and gamma diversity from monotonic declines with elevation to unimodal distributions with a peak around 600 m. Olff & Ritchie ([46]) suggest that grazing alters diversity by affecting the balance between extinction and colonization. At our more productive lower-elevation sites, grazing maintains diversity by creating microsites for species to establish through trampling (increased colonization) and reducing competition for light by removing biomass from competitive species and preventing the build-up of deep litter layers (reduced extinction). At the higher elevation Hartfell site there was no overall significant effect of grazing, but grazing did alter the change in diversity with elevation where the ungrazed SoF experienced a greater decline in diversity than the grazed SoF. Here the higher diversity on ungrazed plots from 600-750 m elevation might be explained by grazing increasing extinction rates in locations already subject to more stressful abiotic conditions (e.g., low soil fertility, cool temperatures). The higher diversity in grazed plots at the highest elevation (800 m) is, however, more difficult to interpret, but it may be that disturbance associated with grazing breaks up the strong dominance of J. squarosus, C. bigelowii and R. lanuginosum and creates microsites for other species to establish.
Our research covers a relatively short period (ca. 3 years) since the restoration process began but nevertheless demonstrates that early monitoring can detect important variation in initial trajectories of vegetation development. The differences we recorded might also be explained by the fact that low-elevations will tend to be more productive and changes following restoration, such as plant growth and the build-up of layers of plant litter, may be faster in such zones. Further monitoring will be needed to determine long-term trajectories with confidence, but one important implication of our work is that the window for facilitating the establishment of absent keystone species, such as Calluna, may be rather limited in more productive locations if more intensive management is to be avoided.
Changes in community composition
Data on the effects of grazing on heathland communities can sometimes appear contradictory. Both Bullock & Pakeman ([13]) and Newton et al. ([43]) revealed a tendency for grazing to reduce the relative abundance of Calluna compared to graminoids in lowland heaths. Nevertheless, managed grazing can form a successful strategy for the restoration of degraded heathland communities. For instance, Grant et al. ([25]) and Common et al. ([15]) showed that cattle grazing can be used to reduce the abundance of N. stricta; Critchley et al. ([16]) found that combined cattle and sheep grazing can be used to reduce the abundance of M. caerulea; and Bullock & Pakeman ([13]) found heavy combined cattle and pony grazing reduced M. caerulea dominance on lowland heaths.
Community structure in our study was principally a function of elevation and site. Although we recorded significant effects of grazing on species diversity, there was no evidence that its removal had significantly altered community structures or initiated a trajectory of development towards a Calluna-dominated habitat. All but one of the community types we identified were characterized by a mixture of grasses and sedges. M. caerulea was at least co-dominant in a number and this has substantial implications for future restoration success. Thus, similarly to previous studies, our research found that for grazing removal alone to initiate Calluna recovery it must be present in the pre-restoration vegetation. V. myrtillus, which is able to regenerate vegetatively via rhizomes, was relatively abundant and appeared to have increased in ungrazed plots. The lack of Calluna regeneration in restored areas can therefore be ascribed to a depauperate seedbank, a lack of a local seed source and intense competition from competitive species. A Vaccinium-dominated community was identified on Hartfell but this shrub was also common on Linshaw Knowe and an alternative shrubland community type may thus develop in some ungrazed areas.
Previous studies on the restoration of upland heaths following over-grazing have had variable success with regards to the establishment of key dwarf shrub species. In many cases the removal or reduction of grazing by sheep has allowed Calluna to re-establish its dominance ([31], [48], [22], [17]) but this has certainly not been true everywhere ([50], [36]), and success thus requires that Calluna be present in the pre-restoration vegetation. On M. caerulea or N. stricta dominated sites success in the restoration of Calluna-dominated communities has generally been associated with cattle grazing, ground disturbance by trampling or rotavation, herbicide application and reseeding ([40], [36], [41]). However, long-term studies suggest that sites that have received minimal levels of disturbance during restoration (i.e., removal of grazing alone) tend to move closer to target conditions ([37]). Differences between studies can be ascribed both to variation in pre-restoration community composition, differences in the season, intensity and stock used in grazing experiments and site edaphic characteristics.
Conclusions
Studies of elevational changes in species diversity have played an important role in defining species’ responses to geophysical variables and understanding patterns of species diversity at multiple scales ([35], [14]). Our results make an important contribution to such work by demonstrating that spatial variation in management intensity can significantly modify such patterns. Grazing has disparate effects on ecosystem structure across elevational ecotones, and this must be considered when applying landscape-scale changes to management. At lower elevations disturbance plays a key role in moderating the dominance of competitive grasses and an alternative to grazing (e.g., herbicide, mechanical disturbance) will be required to prevent their dominance. At higher elevations grazing removal is to the benefit of species richness and diversity. Changes in upland vegetation following grazing removal are slow and we did not detect significant differences in community composition at our site three years after grazing was removed. Our data show changes in diversity can occur quickly and thus appear to be a valuable indicator of potential longer-term trajectories. Restoration of forest, woodland-edge and heathland ecosystems in sites degraded by over-grazing is a significant challenge in the British uplands, but simply removing or reducing grazing pressure may not always provide desirable outcomes. Managers proposing significant modification of upland landscapes should carefully track changes in species diversity in order to detect potentially undesirable trends.
Acknowledgements
This study was funded by the Borders Forest Trust (BFT), thanks to Phil Roe and Nicola Hunt for their support for the study. We also thank members of the BFT Corehead and Devil’s Beeftub Steering Group, and Carol Woolliams in particular, for providing background knowledge. Paul Johnson (University of Glasgow) and Jon Bakker (University of Washington) provided statistical advice. We are grateful to two anonymous referees for their comments.
Author contributions: GMD conceived and designed the research and completed the statistical analyses; JB collected the field data and analysed species richness and diversity. GMD and JB wrote the manuscript.
References
Gscholar
Gscholar
CrossRef | Gscholar
Gscholar
Supplementary Material
Authors’ Info
Authors’ Affiliation
School of Environment and Natural Resources, The Ohio State University, Kottman Hall, 2021 Coffey Road, 43210 Columbus, OH (USA)
Solway Centre for Environment and Culture, University of Glasgow, Rutherford/McCowan Building, Crichton University Campus, Dumfries DG1 4ZL (Scotland)
Corresponding author
Paper Info
Citation
Davies GM, Bodart J (2015). Changes in vegetation diversity and composition following livestock removal along an upland elevational gradient. iForest 8: 582-589. - doi: 10.3832/ifor1557-008
Academic Editor
Arthur Gessler
Paper history
Received: Jan 12, 2015
Accepted: Mar 18, 2015
First online: Apr 22, 2015
Publication Date: Oct 01, 2015
Publication Time: 1.17 months
Copyright Information
© SISEF - The Italian Society of Silviculture and Forest Ecology 2015
Open Access
This article is distributed under the terms of the Creative Commons Attribution-Non Commercial 4.0 International (https://creativecommons.org/licenses/by-nc/4.0/), which permits unrestricted use, distribution, and reproduction in any medium, provided you give appropriate credit to the original author(s) and the source, provide a link to the Creative Commons license, and indicate if changes were made.
Web Metrics
Breakdown by View Type
Article Usage
Total Article Views: 27901
(from publication date up to now)
Breakdown by View Type
HTML Page Views: 20858
Abstract Page Views: 1050
PDF Downloads: 4735
Citation/Reference Downloads: 28
XML Downloads: 1230
Web Metrics
Days since publication: 3384
Overall contacts: 27901
Avg. contacts per week: 57.71
Article Citations
Article citations are based on data periodically collected from the Clarivate Web of Science web site
(last update: Nov 2020)
Total number of cites (since 2015): 7
Average cites per year: 1.17
Publication Metrics
by Dimensions ©
Articles citing this article
List of the papers citing this article based on CrossRef Cited-by.
Related Contents
iForest Similar Articles
Research Articles
Tree encroachment dynamics in heathlands of north-west Italy: the fire regime hypothesis
vol. 3, pp. 137-143 (online: 27 September 2010)
Technical Notes
Goat grazing as a wildfire prevention tool: a basic review
vol. 7, pp. 260-268 (online: 26 March 2014)
Short Communications
The Polish landscape changing due to forest policy and forest management
vol. 2, pp. 140-142 (online: 30 July 2009)
Research Articles
Post-fire recovery of the plant community in Pinus brutia forests: active vs. indirect restoration techniques after salvage logging
vol. 11, pp. 635-642 (online: 04 October 2018)
Research Articles
Agronomic methods for mountain grassland habitat restoration for faunistic purposes in a protected area of the northern Apennines (Italy)
vol. 9, pp. 490-496 (online: 07 January 2016)
Research Articles
Scale dependency of the effects of landscape structure and stand age on species richness and aboveground biomass of tropical dry forests
vol. 16, pp. 234-242 (online: 23 August 2023)
Review Papers
Large-scale effects of forest management in Mediterranean landscapes of Europe
vol. 6, pp. 342-346 (online: 29 August 2013)
Research Articles
How do urban dwellers react to potential landscape changes in recreation areas? A case study with particular focus on the introduction of dendromass in the Hamburg Metropolitan Region
vol. 7, pp. 423-433 (online: 19 May 2014)
Review Papers
Shaping the multifunctional tree: the use of Salicaceae in environmental restoration
vol. 6, pp. 37-47 (online: 21 January 2013)
Research Articles
Approaches to classifying and restoring degraded tropical forests for the anticipated REDD+ climate change mitigation mechanism
vol. 4, pp. 1-6 (online: 27 January 2011)
iForest Database Search
Search By Author
Search By Keyword
Google Scholar Search
Citing Articles
Search By Author
Search By Keywords
PubMed Search
Search By Author
Search By Keyword