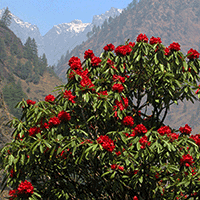
Influence of tree water potential in inducing flowering in Rhododendron arboreum in the central Himalayan region
iForest - Biogeosciences and Forestry, Volume 9, Issue 5, Pages 842-846 (2016)
doi: https://doi.org/10.3832/ifor1525-008
Published: May 06, 2016 - Copyright © 2016 SISEF
Research Articles
Abstract
Rise in temperature has been reported as the principal cause of variation in flowering phenology in several tree species around the globe. In this study, we hypothesized that not only temperature but also rainfall periodicity, soil moisture and the related changes of twig water potential (ψ) in winter and early spring are important drivers of bud expansion and flowering in Rhododendron arboreum in central Himalayas. To this purpose, phenological and physiological variables (flowering time, flower bud size and twig water potential) were monitored over two years in a wild population of R. arboreum (Uttarakhand, India) and related with environmental variables (rainfall, soil moisture and temperature). Results showed that a rise in twig ψ to -0.7MPa, one week after moderate winter precipitation resulted in flower bud enlargement. In both years flowering was triggered after twig ψ reached the threshold of -0.5 Mpa, though the starting date differed between years. Floral bud size was correlated positively with twig ψ (r = 0.43, df =162, p < 0.001) and soil moisture (r = 0.61, df = 71, p < 0.001), while temperature did not influence flower bud size, soil moisture and twig ψ. Flower bud size increment was related with increase in twig ψ and soil moisture. Based on our results, we concluded that water availability plays an important role in inducing flowering in R. arboreum.
Keywords
Rhododendron, Global Warming, Flowering, Water Potential, Himalaya
Introduction
The extent to which plant species are able to adapt to climate change is a central question for ecologists around the globe and is of great importance for both conservation and resource management. Over the past century, earth warmed up by 0.6 °C and most scientists agree that a doubling of atmospheric CO2 concentration would rise the global temperature by 3 0C by the end of the next century ([14]). Continued emission of green house gases at the current or accelerated rates would cause further warming during the 21st century, with an expected increase of global temperature between 2.6 0C and 4.8 °C ([13]). The Indian Himalayan region is particularly vulnerable to the impact of global warming ([31]). Erratic rainfall patterns and long droughts may especially limit plant growth, in a highly seasonal climate. There is significant evidence that climate change has already induced changes in phenological phases of several species ([12], [37], [9], [26], [11], [17], [8]). Flowering time, its duration, and synchrony may affect the interactions of plants with their pollinators and seed dispersers ([1]). Phenological timing is important to the reproductive success and growth of plants ([16], [6], [1]).
Water is one of the most important climatic factor affecting the growth and distribution of terrestrial plants. Flower bud expansion and shoot growth involving cell expansion are known to be inhibited by even moderate water deficit ([4]). Trees in seasonal climates often experience major water deficiency, and their physiology and phenology reflect the seasonality of moisture deficits ([32]).
The forests of Central Himalayas are dominated by oak and pine species ([30]). Water relations of these forests have been investigated by Zobel & Singh ([40]), Tewari ([34]), Zobel et al. ([39]), Poudyal et al. ([21]) and Singh et al. ([32]), while their phenology was studied by Ralhan et al. ([22]), Pangtey et al. ([19]), Rawal et al. ([24]) and Negi ([18]). Rhododendrons (Ericaceae) are under-canopy forest tree species widely distributed around the globe. There are about 1000 species of Rhododendron in the world, of which only 6 occur in the Indian central Himalayan region ([24]). Rhododendron arboreum Sm. is an early successional, evergreen species growing between 1200 m a.s.l. up to the timber line in the Pinus roxburghii and Quercus forests of the Himalayan region. R. arboreum is perhaps amongst the most studied species in the recent past due to evident changes in its flowering phenology, mainly attributed to global warming ([23], [29], [10]).
In this study, the phenological changes of R. arboreum have been studied from a different perspective, particularly by considering the relationship between the periodicity of rainfall and the twig water potential (ψ). To our best knowledge, no study have related the effect of water availability on the physiology and phenology of this species in the Indian Himalayan region. We hypothesized that tree water potential and soil moisture could be important drivers of bud expansion and flowering in R. arboreum. We gathered data on temperature and rainfall, soil moisture, twig water potential, flower buds size and flowering time during the winter and early spring (the probable time of flowering in R. arboreum) over a two year period.
Materials and methods
The study area is located in the central Himalayan region (Uttarakhand province, India) between 29° 23′ 15.1″ and 29° 23′ 17.5″ N of latitude and 79° 30′ 31.0″ and 79° 30′ 38.3″ E of longitude, at an elevation ranging between 1760 and 1780 m a.s.l. Monsoon climate is prevalent in the study area. Meteorological data were collected from the Arya Bhatt Research Institute of Observational Sciences (ARIES), Nainital, located at a distance of 2 km from the study site at 1900 m a.s.l. Mean annual temperature is 14.8 °C, with a mean minimum temperature of the coldest month (January) of 8.2 °C and a mean maximum temperature of the warmest month (June) of 21.9 °C. The annual rainfall is 1267 mm on average (Fig. 1). The rocks of the study area are metamorphosed and soils are sandy in nature ([35], [2]). Anthropogenic disturbances in the study area are moderate and homogenous in terms of fire, lopping and grazing. Fire has not occurred in the area during the last 3-4 years and a herd of 4-5 cattle was seen grazing at the study site on every sampling date. However, no lopping scars were detected on branches of R. arboreum trees.
Fig. 1 - Monthly variations in temperature and rainfall pattern during study period. Bar represent rainfall, lines with solid triangles minimum temperature and solid squares maximum temperature. Arrows indicate the experimental period.
A transect of 400 m long and 50 m wide was established at the study site on a slope ranging between 15° and 23° with south-eastern aspect. Tree layer vegetation was analyzed by placing twenty random quadrats of 10 × 10 m ([27]). Tree density was analyzed following the method proposed by Curtis & McIntosh ([7]) and the basal area was estimated as c2/4π, where c is circumference at breast height (1.37 m above the ground).
The survey on tree flowering phenology was conducted for two consecutive years (2012-2014) between December and March (when trees flower). Forty trees of R. arboreum (29% of the whole population) were selected and marked within the transect: 13 at both ends of transect and 14 in the middle. All selected individuals were approximately of similar height (range: 4.0-6.7 m) and diameter (range: 8.3-10-7 cm).
Data collection of flower bud size and twig water potential (ψ) started in both years by the third week of December and completed with the opening of floral buds (March). Measurements were taken on 18 trees (13% of the population) out of the 40 marked individuals of R. arboreum (6 each at base, middle and top of transect). Twig ψ was measured using a pressure chamber (Model 1000, PMS Instrument Co., Albany, OR, USA - range 70 bars) by taking twig samples from 2-3 m above the ground. Pre-dawn tree ψ (05:30-07:00 am) was measured on 15-cm long leaf bearing twig (3 twigs per tree - [39]). Five floral buds per tree were labeled in December of both years to measure changes in bud size, estimated as the product of bud length and bud width (mm2) measured with a digital caliper (Model CD-6”CS, accuracy +0.02mm - Mitutoyo Co., New Delhi, India). Pre-dawn twig ψ and bud size were measured on the same day and tree. Six measurements between December 2012 and March 2013 (hereafter referred as Yr1) and four measurements between December 2013 and January 2014 (hereafter: Yr2) were taken, as flowering started earlier in Yr2. Flowering pattern was also observed from marked trees and observation on flowering was taken after a three-day interval from the opening of the first flowering bud. Bud opening was considered as the date when dormant flower bud was observed to be splitting ([3]). Result was expressed in terms of percentage of flowering trees. Soil moisture was estimated on a fresh weight basis in December, after the prolonged drought of 3 months and one week (hereafter: Wk) after winter rainfall of January in both the years. After the end of the monsoon season (mid-September), there was very little (<2 cm) rainfall till January. To determine soil moisture, composite soil samples were prepared from three replicates, each of 10 g from the top 30 cm layer, taken from below the canopy of each tree marked for twig ψ measurement. Data were expressed in terms of mean and standard error of mean.
Paired t-test was used to test for difference between twig ψ and floral bud increment among successive collection dates. Linear regression was applied to estimate the relationship between twig ψ, soil moisture and flower bud size; between soil moisture and flower bud size; between twig ψ and flower bud size with maximum, minimum and mean temperature. Data analysis was carried out using the software Microsoft Excel®. ANOVA was used to test the effect of twig ψ, temperature and their interaction on flower bud size change following Snedecor & Cochran ([33]).
Results
Density of R. arboreum was 70 trees ha-1 in the transect. Other associated species (all evergreen) were Pinus roxburghii, Quercus leucotrichophora and Myrica esculenta (Tab. 1). After the last monsoon rains of September, soils had become dry till December in both years, as no significant rainfall occurred during this period. Only sporadic rainfall (<2cm), spread over a three-month period had occurred from September end to Wk 3, January. Soil moisture in Wk3 December was 13.2 ± 0.5 % in Yr1 and 11.3 ± 1.4 % in Yr2. After the rainfall, soil moisture increased to 27.8 ± 0.5 % in Wk1 February Yr1 and 21.9 ± 0.4 % in Wk3 January Yr2 up to 30 cm depth.
Tab. 1 - Main characteristics of tree species found in the studied transect.
Species | Density (trees ha-1) |
Basal area (m2 ha-1) |
---|---|---|
Rhododendron arboreum | 70 | 2.87 |
Quercus leucotrichophora | 290 | 15.19 |
Pinus roxburghii | 300 | 15.17 |
Myrica esculenta | 110 | 4.73 |
Yr1 ud size and flowering
Average pre-dawn twig ψ was -0.88 ± 0.03 MPa during Wk3 December 2012 and further declined to -1.45 ± 0.08 MPa in Wk1 February 2013. One week after rainfall, twig ψ increased to -0.56 ± 0.06 MPa in Wk3 February (Fig. 2a). The t-test indicated that twig ψ started declining significantly after Wk1 January (t = -3.99, df = 17, p < 0.05) and continued to decline until Wk1 February, rising significantly in Wk3 February (t = 8.9, df = 17, p < 0.05), one week after the rainfall (Fig. 3). Moderate rainfall (12.4 cm) occurred 135 days after the end of monsoon season (last rainfall in September 2012), in Wk2 February. Weekly mean temperature ranged between 8.0 and 9.6 0C from December 2012 to February end 2013 and then rose rapidly (Fig. 3). Flower bud size increased from 74.6 ± 1.6 mm2 in Wk3 December to 145.2 ± 2.4 mm2 in Wk3 February (Fig. 2b). The t-test revealed that bud size remained more or less constant till Wk3 January, increased significantly between Wk3 January and Wk1 February (t = 4.8, df = 17, p < 0.05) and the increment was more rapid between Wk1 February and Wk3 February (t = 13.86, df =17, p<0.05). Flowering started in Wk1 March and peaked (>90% trees had flowers) by Wk3 March.
Fig. 2 - (a) Pre-dawn twig ψ of R. arboreum in different weeks during flowering time in Yr1 and Yr2. Each dot represents a week of measurement of pre-dawn ψ. Yr1 denotes measurement weeks between December 2012 and March 2013 and Yr2 weeks between December 2013 and February 2014. D3 denotes Wk3 of December, J1 for Wk1 January, J3 for Wk3 January, F1 for Wk1 February, F3 for Wk3 February and M1 for Wk1March. (b) Floral bud size (mm2) increment in R. arboreum at different weeks during flowering time in Yr1 and Yr2. Each dot represents the week of bud size measurement.
Fig. 3 - Weekly variations in temperature and rainfall during the study period. Light shaded bars represents rainfall in Yr1 and dark shaded bar represents rainfall in Yr2. Solid line represents temperature of Yr1 and dashed represents temperature of Yr2. Weeks are as per Gregorian calendar (stating from December 1). Other labels are as in Fig. 2.
Yr2 ud size and flowering
Average pre-dawn twig ψ was -1.17 ± 0.08 MPa during Wk3 December 2013, then declined significantly to -1.47± 0.07 MPa in Wk1 January 2014 (t = -3.05, df = 17, p < 0.05). One week after rainfall, twig ψ increased significantly to -0.46± 0.02 MPa in Wk1 February (t = 13.65, df = 17, p<0.05 - Fig. 2a). Rainfall (>7cm) occurred 105 days after the end of monsoon in Wk3 January. Weekly mean temperature from December 2013 ranged between 8.0 and 9.3 0C until Wk2 of March 2014, and then rose sharply (Fig. 3). Floral bud size increased from 73.3 mm2 in Wk3 December to 144.5 ± 7.6 mm2 in Wk1 February (Fig. 2b). The t-test revealed that bud size remained more or less constant up to Wk3 January and then increased significantly (t = 6.53, df =17, p < 0.05). Flowering started from the last week of January and peaked in Wk2 February, when more than 60% of trees had flowers.
No significant change in flower bud size was observed over the drought months, though buds started to expand in size following monsoon rains and after moderate precipitation in Wk1 February Yr1 and Wk3 January in Yr2. Bud size started to increase one week after rainfall in both years but the increment was more rapid in Yr2. ANOVA revealed a significant effect of twig ψ on flower bud size (F[5, 126] = 60.79, p<0.0001), while the effect of temperature was not significant (F[5, 126] = 0.51, p = 0.40). However, interaction of twig ψ × temperature was also significant (F[25, 126] = 5.57, p < 0.01). Floral bud size and soil moisture were positively correlated with twig ψ (r = 0.43, df = 162, p<0.001 and r = 0.64, df = 71, p<0.001, respectively - Fig. 4a, Fig. 4b). Soil moisture was also positively correlated with flower bud size (r = 0.69, df = 71, p < 0.001 - Fig. 4c). Contrastingly, fortnightly maximum temperature (r = 0.03, df = 8, p > 0.05), minimum temperature (r = 0.41, df = 8, p > 0.05) and average temperature (r = 0.05, df = 8, p > 0.05) were not significantly correlated with twig ψ. Similarly, none of the three parameters above were significantly correlated with flower bud size (r = 0.45, o.2 and 0.3, respectively; df = 8, p > 0.05).
Fig. 4 - Correlations between (a) twig ψ and flower bud size; (b) twig ψ and soil moisture; and (c) flower bud size and soil moisture of R. arboreum in central Himalaya.
Discussion
In dry environments, heterogeneity and periodicity of water availability have been demonstrated as being crucial factor in determining the phenological rhythm of tree communities and populations ([15], [28]). Twig ψ plays an important role in bud emergence and expansion ([4]). Indeed, Himalayan trees suffer significant water stress, though its intensity differs among different forest types ([39], [18]). Twig ψ of R. arboreum had declined to -1.5 MPa during the post monsoon period (between October and January) and started to increase 7-8 days after precipitation in both years. In Yr1 preciitation was 5.3 cm in Wk1 February and 7.0 cm in Yr2 at the start of Wk3 January (Fig. 3). Rainfall caused an increase of twig ψ up to -0.7 MPa, determining a significant enlargement of floral bud size, and a further increase in twig ψ to -0.5 MPa triggered flowering in R. arboreum (Fig. 4a). In tropical trees bud growth is blocked as long as stem ψ is low, while flowering and bud break occurs only when the stem ψ increases above -0.2 MPa ([4]). In an artificial watering experiment in Tabebwa ochracea, a tropical tree species, flower buds’ size increased within 2 days of watering and were in full bloom after 5-7 days ([5]). Some tree species are able to rehydrate active tissues before leaf flushing by withdrawing stored stem water ([21]). Significant increase in soil moisture percentage after precipitation is quite obvious and consistent with other studies. In a study on forests of Kumaun Himalaya, Tewari ([34]) found that soil ψ (60 cm depth) remains high up to autumn because of the continuing effect of the concentrated monsoon rainfall and declines rapidly in subsequent winters to an extent that trees may need to draw water from deeper soils.
Studies of Ranjitkar et al. ([23]), Gaira et al. ([10]) and Shah et al. ([29]) focused on the role of global warming in inducing flowering in R. arboreum. Contrary to their findings, we did not observe the influence of temperature on bud size increment as well as twig ψ in both years. This could be due to differences in elevation between the investigated sites. Indeed, our study site was located at the lower altitudinal range of R. arboreum where the temperature is sufficiently warm even during winters (6.0-8.0 0C on average) for flowering to occur after precipitation. Contrastingly, studies of Ranjitkar et al. ([23]) and Shah et al. ([29]) were carried out at higher elevation (above 2000 m a.s.l.) where temperature could be limiting. However, temperature data given in these studies have been collected from lower boundary of the species’ altitudinal range (1700 and 2000 m) and therefore should not fully represent the temperature of the entire elevation range. On-site meteorological data collection is very difficult in Himalayan forests, due to the intense human presence that hinder the possibility to leave the instruments in the field.
Temperature is considered as an important driver of plant phenology in temperate and boreal climate of Europe and North America ([20], [25], [36], [38]), where soil water availability is not a limiting factor. Our study area lies in a warmer climate with a strongly seasonal precipitation and drought periods often occurring at the beginning of tree growth, thus representing a limiting factor for tree phenology.
Our results clearly revealed that flower bud enlargement occurred in both years only after winter precipitation, with trees flowering when predawn twig ψ increases above the threshold of -0.5 MPa. Variation in winter rainfall pattern together with marginal rise in minimum temperature during flowering time could be responsible for variation in flowering phenology between the years.
Conclusions
Global warming is thought to dramatically affect phenological shifts and flowering worldwide. The results of this study indicates that tree water potential and soil moisture have an important role in inducing bud enlargement and flowering in R. arboreum. Indeed, temperature alone did not trigger flowering in the studied species. Instead, our results indicate that the increase in soil moisture and twig water potential are a prerequisite for initiation of flower expansion in this species. However, studies based on more sites and years using on-site temperature data are required for better understanding the factors underlying the observed phenological changes in R. arboreum.
Acknowledgements
This study was funded by the Science and Engineering Research Board (SERB), New Delhi, India (Grant. No. SERB/SR/SO/PS/ 08/2010). AT and JB contributed to data analysis and drafted the manuscript. AM carried out field measurements. The authors thank the head of the Department of Forestry and Environmental Sciences for providing the departmental services. We are thankful to Prof. S. P. Singh, FNA, for the assistance in restructuring the manuscript and to Prof. Madhu Joshi, Department of English, KU Nainital (India) for language improvement. Finally, the authors are grateful to anonymous reviewers whose comments helped in improving the manuscript.
References
Gscholar
Gscholar
Gscholar
Gscholar
CrossRef | Gscholar
Gscholar
Gscholar
Gscholar
Gscholar
Gscholar
Gscholar
Gscholar
Gscholar
Authors’ Info
Authors’ Affiliation
Jitendra Bhatt
Amit Mittal
Department of Forestry and Environmental Science, Kumaun University, Nainital 263002 (India)
Corresponding author
Paper Info
Citation
Tewari A, Bhatt J, Mittal A (2016). Influence of tree water potential in inducing flowering in Rhododendron arboreum in the central Himalayan region. iForest 9: 842-846. - doi: 10.3832/ifor1525-008
Academic Editor
Silvano Fares
Paper history
Received: Dec 11, 2014
Accepted: Dec 07, 2015
First online: May 06, 2016
Publication Date: Oct 13, 2016
Publication Time: 5.03 months
Copyright Information
© SISEF - The Italian Society of Silviculture and Forest Ecology 2016
Open Access
This article is distributed under the terms of the Creative Commons Attribution-Non Commercial 4.0 International (https://creativecommons.org/licenses/by-nc/4.0/), which permits unrestricted use, distribution, and reproduction in any medium, provided you give appropriate credit to the original author(s) and the source, provide a link to the Creative Commons license, and indicate if changes were made.
Web Metrics
Breakdown by View Type
Article Usage
Total Article Views: 19581
(from publication date up to now)
Breakdown by View Type
HTML Page Views: 14577
Abstract Page Views: 900
PDF Downloads: 3042
Citation/Reference Downloads: 17
XML Downloads: 1045
Web Metrics
Days since publication: 3004
Overall contacts: 19581
Avg. contacts per week: 45.63
Article Citations
Article citations are based on data periodically collected from the Clarivate Web of Science web site
(last update: Nov 2020)
Total number of cites (since 2016): 2
Average cites per year: 0.40
Publication Metrics
by Dimensions ©
Articles citing this article
List of the papers citing this article based on CrossRef Cited-by.
Related Contents
iForest Similar Articles
Research Articles
Links between phenology and ecophysiology in a European beech forest
vol. 8, pp. 438-447 (online: 15 December 2014)
Review Papers
Wood anatomy of boreal species in a warming world: a review
vol. 13, pp. 130-138 (online: 09 April 2020)
Research Articles
Use of δ13C as water stress indicator and potential silvicultural decision support tool in Pinus radiata stand management in South Africa
vol. 12, pp. 51-60 (online: 24 January 2019)
Research Articles
Diversity, distribution and vegetation assessment in the Jahlmanal watershed in cold desert of the Lahaul valley, north-western Himalaya, India
vol. 3, pp. 65-71 (online: 17 May 2010)
Research Articles
Spatial and temporal variation of drought impact on black locust (Robinia pseudoacacia L.) water status and growth
vol. 8, pp. 743-747 (online: 18 June 2015)
Research Articles
Testing a dual isotope model to track carbon and water gas exchanges in a Mediterranean forest
vol. 2, pp. 59-66 (online: 18 March 2009)
Research Articles
Climate impacts on tree growth in a Neotropical high mountain forest of the Peruvian Andes
vol. 13, pp. 194-201 (online: 19 May 2020)
Research Articles
Early flowering and genetic containment studies in transgenic poplar
vol. 5, pp. 138-146 (online: 13 June 2012)
Research Articles
Evaluation of fast growing tree water use under different soil moisture regimes using wick lysimeters
vol. 6, pp. 190-200 (online: 08 May 2013)
Research Articles
Matching seedling size to planting conditions: interactive response with soil moisture
vol. 12, pp. 220-225 (online: 25 April 2019)
iForest Database Search
Search By Author
Search By Keyword
Google Scholar Search
Citing Articles
Search By Author
Search By Keywords
PubMed Search
Search By Author
Search By Keyword