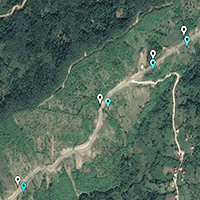
Influences of Black Locust (Robinia pseudoacacia L.) afforestation on soil microbial biomass and activity
iForest - Biogeosciences and Forestry, Volume 9, Issue 1, Pages 171-177 (2015)
doi: https://doi.org/10.3832/ifor1410-007
Published: Feb 16, 2015 - Copyright © 2015 SISEF
Short Communications
Abstract
Black locust is a tree species considered suitable for afforestation in Turkey because of its rapid growth and ability to fix atmospheric nitrogen in disturbed soil ecosystems. Quantitative indicators of soil health and quality can be usefully derived from a data set of soil physical, chemical and microbial characteristics. In this study changes in soil characteristics after afforestation with black locust were assessed by comparing several afforestation sites with control (no vegetation) sites randomly chosen along the roadside in Ulus-Bartin, the western Black Sea region (Turkey). Results showed that some physical and chemical characteristics of the soil (soil bulk density, clay content, soil organic C and total N) were higher at the afforestation sites as compared with the control sites. Similarly, afforestation sites showed higher values for mean soil microbial biomass C (afforestation: 311.97 µg g-1;control: 149.68 µg g-1) and N (afforestation: 43.07 µg g-1; control: 19.21 µg g-1), and basal respiration (afforestation: 0.303 µg CO2-C g-1 h-1; control: 0.167 µg CO2-C g-1 h-1). However, the mean metabolic quotient (qCO2) assessed at the control sites was higher (1.47 mg CO2-C g-1 Cmic h-1) than that observed the afforestation sites (0.96 mg CO2-C g-1 Cmic h-1), likely due to difficulties in the utilization of organic substrates by the microbial community. In addition, the correlation between the qCO2 and Cmic/Corg percentages was negative (r = - 0.586, P < 0.01) in both sites. Our results indicated that afforestation with black locust could be advantageous, not only for soil improvement and regeneration, but also for sustainable land management.
Keywords
Basal Respiration, Cmic/Corg Percentage, Cmic/Nmic Ratio, Metabolic Quotient (qCO2), Nitrogen Fixation
Introduction
Soil health and quality may be successfully monitored through the use of two microbial indexes: (i) the microbial biomass, which is defined by the living component of soil organic matter, excluding the macrofauna and plant roots; and (ii) soil microbial respiration (basal respiration), which is described by the respiration without the addition of an organic substrate to soil ([33], [1], [53]). The microbial biomass is small and labile as compared with other ecosystem components; however, because of its key role in the mineralization of nutrients such as C, N, P and S, it is considered a reliable indicator of changes in soil characteristics ([33], [48], [9]). Balota et al. ([10]) report that changes in the microbial properties of soil may lead to important changes in soil quality, thus affecting nutrient cycling and plant development. Haris ([30]) clearly affirmed that the microbial community serves as an indicator of the re-establishment of connections between ecological functions of the biota in disturbed ecosystems. Despite the large variations in mineralogy, soil texture and land use type, extreme environments usually show low organic matter and very low microbial biomass contents, which indicate poor soil quality and limited site productivity ([53], [29]).
Soil basal respiration is a well-established parameter to be measured through the decomposition process, and it is defined as the overall activity or energy spent by the indigenous microbial pool ([4], [53]). Insam et al. ([31]) argued that soil basal respiration reflects the availability of slow-flowing C for microbial permanence and is a measure of basic turnover rates in soil.
Robinia pesudoacacia L. (black locust or false acacia) is a deciduous, broad-leaved, light-demanding, medium-sized pioneer tree ranging in height 20-25 m. Black locust is regarded as an invasive, non-indigenous species in many parts of Europe, such as Germany, Italy and Turkey ([7], [22]). It is well-adapted to grow on different types of soil and environmetal conditions, though it avoids compacted or wet soils. Indeed, its demand for aerated soil is the reason why black locust can be primarily found in disturbed sites from poor to fertile soils ([52], [22]). Black locust shows a rapid growth and adaptability, for it has been utilized for soil conservation or fuel wood, as well as for early reforestation of barren soils at disturbed sites ([40], [38], [44]). However, in Turkey it has been long cultivated as an ornamental plant and it can be currently found in schoolyards, roadsides or other marginal lands ([7]). This tree species is characterized by an extensive root system ([69]) nodulated by diverse nitrogen-fixing Rhizobium communities, providing the host with an advantage over native species on poor soils, and increasing thoroughly the soil nitrogen content ([44], [49], [22]). For these reasons, black locust is presumed to be a favorable tree species for soil reclamation and regeneration of disturbed sites, such as roadsides and former mine sites.
Although many studies has been conducted on the influences of black locust on soil physicochemical and nutritional properties ([23], [55], [61], [68]), the reclamation of soil properties by black locust is still not well understood ([38], [66]), especially in terms of microbial biomass and activity.
The purpose of the study was to investigate the effect of afforestation with black locust on: (1) soil physical and chemical properties, such as soil moisture, bulk density, soil organic C and total N content; (2) soil microbial biomass C (Cmic) and N (Nmic); and (3) soil microbial activity as basal respiration.
Materials and ethods
Site description
The study was conducted at a black locust 8-years-old afforestation site located in Ulus-Bartin, the western Black Sea region of Turkey (32° 45′ 47″ - 32° 47′ 06″ E, 41° 37′ 34″ - 41° 38′ 02″ N). Based on the Thornthwaite method ([56]), the study site has a humid, mesothermal oceanic-like climate, with little or none water summer deficit. According to climatological data over the past 30 years ([57]), the average annual air temperature is 9.1 °C, the average temperature of the coldest month (January) is 3.0 °C, and the average temperature of the warmest month (July) is 16.2 °C. Average annual precipitation is 1385.1 mm, with about 35% of rainfall occurring from May to September. Relative humidity is nearly 80%. The main bedrock of the study site is calcareous limestone, with a stony and coarse texture.
Two-years-old seedlings of black locust were planted in 2008 along the roadsides of the Ulus-Pinarbasi road. The study was carried out in July 2013, when the black locust trees were eight years old. Tree diameter at breast height ranged from 10 to 18 cm, while tree height varied between 5 and 8 m. As a control site (with no afforestation), an area located on the opposite side of the road and lacking in any tree species and vegetation was selected. Mean elevation at the study site was 775 m a.s.l., ranging between 650 and 900 m, with a south-southeast aspect and a slope of 40-50%.
Six sampling locations (named 1 to 6) were established along the roadsides (Fig. 1), with a distance of 2.2 km between the farthest locations (1 and 6). The distance between points 1 and 2 was 750 m; 2-3: 400 m; 3-4: 250 m; 4-5: 250 m; 5-6: 550 m. At each location, soil sampling was carried out on both sides of the road: the control, vegetation-free points (1c to 6c) on one side, and the afforested points (1a to 6a) on the opposite side. The distance from the road of both the control (c) and the afforestation points (a) was approximately 4-5 m.
Fig. 1 - Sampling sites in the study area (Ulus-Pinarbasi road, Ulus-Bartin, western Black Sea region, Turkey).
Soil sampling
To determine physical and chemical properties of the soil, samples of topsoil (0-6.5 cm depth, 8.1 cm in diameter) were randomly collected at both control and afforestation points, totaling 12 sampling points. The same number of samples were also taken from topsoil at the same locations for microbial analysis after removing stones, plant and root debris. A soil depth of 0-6.5 cm was chosen for sampling, since this layer was considered to be more susceptible to any change (e.g., afforestation) as compared with lower layers.
Soil samples for microbial analysis were sifted with 2-mm sieves and stored at 4 °C until the measurement of the microbial biomass C and N, and basal respiration. Instead, soil cores for physical and chemical analysis were air-dried, ground and sieved (< 2 mm).
Physical and chemical analyses
The moisture content of soil samples was determined gravimetrically by oven-drying at 105 °C until a constant weight was reached. The hydrometer method was used to calculate the particle size distribution of the soil ([16]). Soil pH was measured in a 1:2.5 soil:water suspension using a pH meter, while the electrical conductivity of the soil was determined on a 1:5 soil:water extract with an electrical conductivity meter. Organic C content of soil samples was estimated using potassium dichromate oxidation, and the total N content was determined by Kjeldahl digestion. The Scheibler calcimeter method was used to measure the CaCO3 content of the soil ([51]). Soil bulk density (g cm-3) was calculated using mass and volume ([14]). The particle density of the soils (g cm-3) was measured using the Pycnometer method and pore space was calculated using bulk and particle densities ([17]).
Biochemical analyses
Microbial biomass C and N were determined by the chloroform fumigation extraction method ([5]). Microbial biomass C (C
mic) was determined by a modified Walkley-Black method ([18], [59]) using the following equation ([59] - eqn. 1):
where KEC refers to the difference in extractable organic C between the fumigated and unfumigated samples, and 2.64 is a proportionality factor used for the biomass C
released by fumigation extraction.
The method described by Brookes et al. ([18]) and Anderson & Ingram ([5]) was used for measuring the microbial biomass N (N
mic), calculated as follows (eqn. 2):
where FN is the difference between N
extracted from fumigated and unfumigated samples. The fraction of microbial biomass N
released by fumigation extraction is 0.54.
Basal respiration was determined by the sodium hydroxide (NaOH) trap method ([1]). The metabolic quotient (qCO2) was calculated as the basal respiration rate (mg CO2-C h-1) g-1 of microbial biomass ([4]).
All the above analyses were performed with two replications, and the data obtained were averaged over replicates and expressed on an oven-dry weight basis.
Statistical analyses
Differences in microbial biomass C and N, basal respiration and the physical and chemical characteristics of the soil between control and afforestation sites were tested through independent samples t-tests (α = 0.05). Correlation analysis was applied to assess the relationships between microbial biomass C with basal respiration, and C
mic/C
org with qCO2. All the analyses were carried out using the statistical package SPSS® ver. 16.0.
Results and iscussion
The results of the comparison of the control and afforestation sites in terms of physical and chemical characteristics of soil are summarized in Tab. 1. No differences (P > 0.05) were found for soil particle density, sand fraction, silt fraction and calcium carbonate content. Soil bulk density was considerably higher (P < 0.05) in the afforestation site as compared with the control site. Conversely, soil pore space was significantly higher (P < 0.05) in the control site than in the afforestation site. It is implied in the literature that any factor influencing soil pore space will also affect bulk density. Obviously, since bulk density relates to the combined volumes of the solids and pore spaces, soils with a high proportion of pore space to solids have also lower bulk densities ([17]). In both control and afforestation sites, the soil was sandy clay loam with 49.29-51.42% sand, 17.55-21.33% silt and 27.25-33.16% clay. The soil at the afforestation site had a relatively higher percentage of soil moisture than the soil at the control site, likely due to the higher clay content and the presence of vegetation in the afforestation site. Such results support the evidence that soil texture (especially clay content) and vegetation are important factors in determining the proportion of soil moisture ([25], [36]).
Tab. 1 - Comparisons of the physical and chemical characteristics of the soil at the control and afforestation sites (0-6.5 cm depth). Values within rows followed by the different letters indicate a significant difference between the control and afforestation sites after independent sample t-test (P < 0.05). Values represent the mean of 12 samples ± standard error.
Soil characteristics | Control site | Afforestation site |
---|---|---|
Bulk density (g cm-3) | 1.54 ± 0.048 a | 1.71 ± 0.056 b |
Particle density (g cm-3) | 2.77 ± 0.01 a | 2.77 ± 0.02 a |
Pore space (%) | 44.58 ± 1.70 a | 38.12 ± 2.21 b |
Sand (%) | 51.42 ± 3.53 a | 49.29 ± 2.46 a |
Silt (%) | 21.33 ± 2.97 a | 17.55 ± 0.88 a |
Clay (%) | 27.25 ± 1.04 a | 33.16 ± 1.91 b |
Soil texture | Sandy clay loam | Sand clay loam |
Moisture content (%) | 9.63 ± 0.46 a | 11.44 ± 0.50 b |
Soil pH (H2O) | 8.43 ± 0.02 a | 8.26 ± 0.04 b |
Calcium carbonate (CaCO3 - %) | 7.52 ± 0.68 a | 7.71 ± 0.59 a |
Electrical conductivity (dS m-1) | 0.92 ± 0.03 a | 1.11 ± 0.03 b |
Organic C (%) | 0.45 ± 0.03 a | 0.64 ± 0.04 b |
Total N (%) | 0.14 ± 0.003 a | 0.17 ± 0.008 b |
Corg/Ntotal ratio | 3.16 ± 0.24 a | 3.92 ± 0.25 b |
The pH of soil in the control site was significantly higher than that of soil in the afforestation site (Tab. 1). Soils at both sites were also medium alkaline, which could be due to calcareous limestone. An obvious difference in soil electrical conductivity (EC) between the studied sites was observed. However, the soils analyzed cannot be classified as saline, since they did not show an EC of 4 dS m-1 or more ([54]). Soil organic C and total N are important factors that contribute to improve the physical properties of soil, and then its productivity. The largest soil organic C and total N amount were detected in the soils sampled at the afforestation sites. Such evidence is reasonably related to their higher clay content ([19]), the presence and diversity of tree species ([46], [55], [36]), the higher input of root exudates and plant residues ([28]), and the chemical composition of litter ([35]). Moreover, the above evidence suggest that the observed improvement of the soil is the result of the afforestation with the black locust, which appears to be primarily responsible for the increase in soil organic C and total N, according to previous reports by Rice et al. ([50]), Keskin & Makineci ([38]) and Yüksek ([66]). We hypothesized that the low C
org/N
total ratio observed at the control sites (3.16 ± 0.24) may be due to the rapid breakdown of nitrogen-containing compounds and intensive carbon mineralization, i.e., the higher degree of decomposition of the organic matter ([67], [24]). On the other hand, the high C
org/N
total ratio found in soils from afforested sites can be explained by higher inputs of soil organic carbon, as revealed by the significantly higher soil organic C content in the afforestation sites compared with the control sites (Tab. 1). Moreover, it has been reported in the literature that when organic residues with a high Corg/Ntotal ratio are added to the soil, a keen competition arises among microorganisms for available soil nitrogen, leaving less nitrogen available for nitrification ([17], [42]).
The mean soil microbial biomass C (C
mic) was nearly 48% higher in the afforestation sites than the control sites (Fig. 2a). At the control sites, C
mic values ranged from 43.89 to 261.95 µg g-1, while the corresponding range for the afforestation sites was 219.42-397.11 µg g-1. Similarly, the mean soil microbial biomass N (N
mic) was approximately 45% higher in the afforestation sites than in the control sites (Fig. 2b), varying from 10.41 to 42.83 µg g-1 and from 11.74 to 98.37 µg g-1 in the control and the afforestation sites, respectively. Hence, the afforestation sites have a higher microbial biomass C and N, and the presence of black locust trees has a significant (P < 0.05) effect on soil C
mic and N
mic (Fig. 2a, Fig. 2b). According to McGill et al. ([43]), an increase in soil microbial biomass could result in the immobilization of soil nutrients, whereas its decrease may lead to nutrient mineralization. Also, it has been suggested that a low microbial biomass in the soil could be a hint of either stress or disturbance ([63]). According to previous studies ([60], [47], [27]), our findings confirm that the nitrogen-fixing black locust tree may contribute to the soil C
mic and N
mic pools, improving the rates of carbon and nitrogen cycling and their availability. Moreover, previous studies reported that black locust could be largely ameliorative by promoting the amount and availability of N in the soil ([55], [49], [66]), improving the quality and structure of soil, and increasing root biomass and soil organic C sequestration ([58], [66]), thus ameliorating the biological properties of soils ([65], [61]). Furthermore, some authors argued that the microbial dynamic pool is attached to the organic residues that supply available C and nitrogen contents in the soil ([62], [8]).
Fig. 2 - Changes in mean soil microbial biomass C (a), soil microbial biomass N (b) and soil basal respiration (c) in the soil at the control and afforestation sites (errors bars represent standard error). Significant differences (P < 0.05) between the control and afforestation sites are indicated by different letters (a, b) after independent sample t
-test (P < 0.05).
Soil basal respiration provides an estimate of the microbial activity, being a proxy of the cycling of organic carbon and organic carbon-bound nutrients (N, S, P) within an ecosystem ([64]). In this study, basal respiration rates of the soil ranged from 0.125 to 0.227 µg CO2-C g-1 h-1 at the control sites and from 0.208 to 0.435 µg CO2-C g-1 h-1 at the afforestation sites, the latter showing values significantly higher than control sites (P < 0.05 - Fig. 2c). Also, basal respiration demonstrated a significant positive correlation with microbial biomass C (Fig. 3a). The high basal respiration rate observed in the soil at the afforestation sites might be due to the high moisture content, organic carbon, microbial biomass, and tree root biomass. Indeed, basal respiration is a well-established parameter to monitor decomposition ([1], [53], [64]), although it is often affected by wide natural fluctuations, depending on soil moisture, substrate availability and soil temperature. Nsabimana et al. ([45]) and Cheng & Xia ([21]) asserted that soil basal respiration was positively correlated with soil organic carbon and microbial biomass, and the availability of suitable substrates (protein, carbohydrate, glucose, etc.) is the main factor influencing the activity of microbial community. Islam & Weil ([32]) and Campos et al. ([20]) argued that high rates of basal respiration can occur either as a result of a large pool of labile C substrates or rapid oxidation of a smaller pool. Thus, high basal respiration may indicate either ecological stress and degradation or a high level of ecosystem productivity. For the above reasons, a more clearly interpretable parameter is the rate of basal respiration per unit of microbial biomass (qCO2 - see below).
Fig. 3 - The relation between the microbial biomass C and basal respiration (a), C
mic/C
org and qCO2 (b) for soils sampled at the control and afforestation sites.
In this study, the ratio C
mic/C
org was higher in the afforestation sites as compared with the control sites, though such difference was not statistically significant (P > 0.05 - Tab. 2). About 2.2% of soil organic carbon is constituted of microbial biomass, a value above the equilibrium threshold proposed by Jenkinson & Ladd ([33]), though the observed variation (0.27-7.0%) was well within the range suggested by Anderson & Domsch ([3]). According to these authors, the C
mic/C
org ratio may be considered a suitable indicator of the soil carbon dynamics, and its variation is regarded as a hint of environmental changes in the soil ([12], [6], [26]). In this study, the high C
mic/C
org ratio found at the afforestation sites suggests suitable environmental conditions for the proliferation of soil microrganisms.
Tab. 2 - Derived microbial indexes calculated for control and afforestation sites (0-6.5 cm depth). Values within rows row followed by the same letter indicate no significant difference between the means (independent sample t-test - P > 0.05). Values represent the mean of 12 samples ± standard error.
Microbial index | Control sites | Afforestation sites |
---|---|---|
C mic/C org (%) |
3.67 ± 0.74 a | 5.17 ± 0.49 a |
C mic/N mic (%) |
8.40 ± 1.05 a | 10.83 ± 1.73 a |
N mic/N total (%) |
1.38 ± 0.21 a | 2.95 ± 0.72 a |
qCO2 (mg CO2-C g-1 C mic h-1) |
1.47 ± 0.28 a | 0.96 ± 0.03 a |
Similarly, the ratio C
mic/ N
mic observed at the afforestation sites was higher than that found at the control sites, though not significantly different (P > 0.05 - Tab. 2). The C
mic/N
mic ratio is frequently used to define the structure of the microbial community: it has been pointed out that a high C
mic/N
mic ratio (range: 7-12) may indicate a predominance of fungi in the soil microbial biomass, whereas a low C
mic/N
mic ratio (range: 3-6) may indicate a predominant proportion of bacteria in the microbial community ([34]). According to the above considerations, fungi may represent the dominant populations in the soil at the studied sites.
The N
mic/N
total ratio exhibited the same pattern observed for C
mic/C
org and C
mic/N
mic (Tab. 2). Based on the scientific literature, a relatively high N
mic/N
total value indicates that the availability of organic nitrogen components is not a limiting factor for microbial biomass ([39]), whereas a low N
mic/N
total indicates a decline in substrate quality ([12]).
The metabolic quotient (qCO2) is defined as the substrate mineralized per unit of microbial biomass carbon ([11]), and it has been extensively used to evaluate ecosystem development, disturbance, or system maturity. Anderson & Domsch ([2]) and Bauhus & Khanna ([13]) reported that a high qCO2 value may be a hint of low available C content and substrate quality for microbial biomass, thereby less biomass could be produced since more carbon is used for respiration, thus reflecting a poor rhizosphere health ([6], [26], [27]). In this study, qCO2 in the control sites was higher (though not significantly) than that observed at the afforestation sites (Tab. 2). Such results may be due to difficulties in the utilization of organic substrates by the microbial community at the control sites, and/or to unsuitable soil conditions. Additionally, a close negative relationship between qCO2 and the C
mic/C
org ratio was found (Fig. 3b), according to previous studies ([13], [37], [15]). Furthermore, several authors have argued that a low qCO2 and a high C
mic/ C
org ratio (Tab. 2) may indicate a more efficient use of organic substrates by microbial biomass ([6]) and higher microbial activity ([41]).
Conclusions
In this study, soil chemical characteristics, soil microbial biomass and activity were compared between control and afforestation sites sampled at 12 locations along the Ulus-Pinarbasi road (western Black Sea region, Turkey). The results showed that black locust trees used in the afforestation has positively affected both soil microbial biomass and activity, improving soil characteristics and promoting its quality and health. Despite the invasive nature of black locust, this species could be preferred in such disturbed sites because of its rapid growth, soil amelioration and resistance to harsh environmental conditions. The results of this study may be considered as a baseline for monitoring the possible future changes in the microbial biomass and activity of soil in black locust afforestation sites.
Acknowledgements
We acknowledge the Ulus Forest Administration and Turkish State Meteorological Service (TSMS) for their assistance and data support throughout the study. We would like to thank two anonymous referees for their helpful comments and suggestions.
References
Gscholar
Gscholar
Gscholar
Gscholar
Gscholar
Gscholar
CrossRef | Gscholar
Gscholar
Gscholar
CrossRef | Gscholar
Gscholar
CrossRef | Gscholar
Gscholar
Authors’ Info
Authors’ Affiliation
Bartin University, Faculty of Forestry, Department of Forest Engineering, Division of Soil Science and Ecology, Bartin (Turkey)
Karadeniz Technical University, Faculty of Forestry, Department of Forest Engineering, Division of Watershed Management, Trabzon (Turkey)
Bartin University, Faculty of Forestry, Department of Forest Engineering, Division of Watershed Management, Bartin (Turkey)
Kahramanmaras Sutcu Imam University, Faculty of Forestry, Department of Forest Engineering, Kahramanmaras (Turkey)
Corresponding author
Paper Info
Citation
Bolat I, Kara Ö, Sensoy H, Yüksel K (2015). Influences of Black Locust (Robinia pseudoacacia L.) afforestation on soil microbial biomass and activity. iForest 9: 171-177. - doi: 10.3832/ifor1410-007
Academic Editor
Gianfranco Minotta
Paper history
Received: Jul 27, 2014
Accepted: Oct 16, 2014
First online: Feb 16, 2015
Publication Date: Feb 21, 2016
Publication Time: 4.10 months
Copyright Information
© SISEF - The Italian Society of Silviculture and Forest Ecology 2015
Open Access
This article is distributed under the terms of the Creative Commons Attribution-Non Commercial 4.0 International (https://creativecommons.org/licenses/by-nc/4.0/), which permits unrestricted use, distribution, and reproduction in any medium, provided you give appropriate credit to the original author(s) and the source, provide a link to the Creative Commons license, and indicate if changes were made.
Web Metrics
Breakdown by View Type
Article Usage
Total Article Views: 25659
(from publication date up to now)
Breakdown by View Type
HTML Page Views: 19487
Abstract Page Views: 1100
PDF Downloads: 3741
Citation/Reference Downloads: 38
XML Downloads: 1293
Web Metrics
Days since publication: 3449
Overall contacts: 25659
Avg. contacts per week: 52.08
Article Citations
Article citations are based on data periodically collected from the Clarivate Web of Science web site
(last update: Nov 2020)
Total number of cites (since 2016): 14
Average cites per year: 2.80
Publication Metrics
by Dimensions ©
Articles citing this article
List of the papers citing this article based on CrossRef Cited-by.
Related Contents
iForest Similar Articles
Research Articles
Effects of altitudinal gradients on leaf area index, soil microbial biomass C and microbial activity in a temperate mixed forest ecosystem of Northwestern Turkey
vol. 10, pp. 334-340 (online: 15 December 2016)
Research Articles
Effect of different dolomitic limestone dosages on soil respiration in a mid-altitudinal Norway spruce stand
vol. 12, pp. 357-365 (online: 05 July 2019)
Research Articles
Seasonal dynamics of soil respiration and nitrification in three subtropical plantations in southern China
vol. 9, pp. 813-821 (online: 29 May 2016)
Review Papers
Separating soil respiration components with stable isotopes: natural abundance and labelling approaches
vol. 3, pp. 92-94 (online: 15 July 2010)
Research Articles
Quantifying forest net primary production: combining eddy flux, inventory and metabolic theory
vol. 10, pp. 475-482 (online: 12 April 2017)
Research Articles
Short-time effect of harvesting methods on soil respiration dynamics in a beech forest in southern Mediterranean Italy
vol. 10, pp. 645-651 (online: 20 June 2017)
Research Articles
Spatial heterogeneity of soil respiration in a seasonal rainforest with complex terrain
vol. 6, pp. 65-72 (online: 07 February 2013)
Research Articles
Day and night respiration of three tree species in a temperate forest of northeastern China
vol. 8, pp. 25-32 (online: 26 May 2014)
Research Articles
Thinning effects on soil and microbial respiration in a coppice-originated Carpinus betulus L. stand in Turkey
vol. 9, pp. 783-790 (online: 29 May 2016)
Research Articles
Effects of nitrogen loading under low and high phosphorus conditions on above- and below-ground growth of hybrid larch F1 seedlings
vol. 11, pp. 32-40 (online: 09 January 2018)
iForest Database Search
Search By Author
Search By Keyword
Google Scholar Search
Citing Articles
Search By Author
Search By Keywords
PubMed Search
Search By Author
Search By Keyword