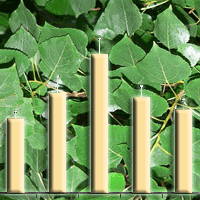
Soil nutrient status, nutrient return and retranslocation in poplar species and clones in northern Iran
iForest - Biogeosciences and Forestry, Volume 6, Issue 6, Pages 336-341 (2013)
doi: https://doi.org/10.3832/ifor0976-006
Published: Aug 29, 2013 - Copyright © 2013 SISEF
Research Articles
Abstract
In this study several same-aged poplar species and clones were selected including Populus deltoides Marsh. CV. “Lux” (P.d “Lux”), Populus deltoides Marsh. CV. “Harvard” (P.d “Harvard”), Populus x canadensis Moench “Triplo” (P.xc “Triplo”), Populus x canadensis Moench “I-45/51” (P.xc “I-45/51”), planted in poplar research station about 20 years ago in the north of Iran, and Populus caspica Bornm. (P. caspica) as endemic and endangered poplar species. Some quantitative characteristics of the trees and soil samples were taken based on completely randomized block design. Samplings of live and senescent leaves were carried out in two-stages, mid-summer during the peak nutritional activities of trees and mid-autumn for senescent leaves. Soil samples were analyzed to determine organic carbon (OC), total nitrogen (N), available phosphorus (P) and exchangeable potassium (K). OC, total of N, P and K were also determined in live and senescent leaves. The results showed that P.d “Harvard” had the highest total height, DBH and diameter of crown compared to the other species and clones. The amount of OC, N and P in soil and live leaves of P.d “Harvard” clone are more than those of the other species and clones. OC, N, P, and K returned by senescent leaves were higher and lower for P.d “Harvard” and P. caspica respectively. P retranslocation for all of the clones and species was higher compared to N and K. The highest retranslocation percentage was observed in P. caspica (N=18.93, P=39.63, K=24.02) and the lowest (N=12.09, P=32.46, K=21.14) in P.d “Harvard” clone. It could be concluded that poplar species and clones have different nutritional requirements and retranslocation capabilities. It appears that P.d “Harvard” clone showed higher quantitative growth it could be beneficial for maintaining the soil nutrient status for successive plantings.
Keywords
Poplar, Retranslocation, Nutrient Return, Soil Properties, Plantation
Introduction
In natural forests and man-made plantations, cycling of nutrient is an important aspect, as significant amounts of nutrients are returned to soil through litter fall and become available for nutrient cycling. Among nutrients, some are used in physiological responses and other stored in different plant organs, or returned to the soil through the litter and then partially absorbed by the root of trees ([5]). Uptake and release of nutrients are important factors at the stand level, because they represent the major fluxes through the system ([22]). Nutrient concentration in plant biomass is the result of the balance between nutrient uptake, plant growth and nutrient retranslocation, and the loss of these processes are likely to be influenced both by plant genetic make-up and soil fertility, as well as other environmental conditions ([10]).
Forest litter fall is the major flux responsible for nutrient transfer to soil ([26]) and the growth and productivity of forest ecosystems depends mainly on the amount, the nature and the rate of decomposition of litter fall ([34]). Tree species can play an important role in nutrient cycling through different properties, such as the amount of litter produced, nutrients release and chemical composition of the litter ([28]). Different tree species involve different nutrient release patterns, which are related to litter quality and seasonal environmental factors ([15]). Tracking nutrient returns through litter fall under different tree species is important to understand the dynamics of soil fertility.
Soil and old leaf nutrient retranslocation, are the primary sources of nutrients in the leaves ([4], [27]) and litter fall nutrient abundance is related to intensity of retranslocation processes in autumn ([26]). The nutrient retranslocation, movement and transfer nutrients from the old leaves to the every year store, is an important process in nutrient dynamics in most ecosystems, especially broadleaf ecosystems ([16], [8]). Retranslocation has been characterized as one of the most important strategies used by trees to conserve nutrients, which consequently influences competition, nutrient uptake, and productivity ([16]). Within the same community, foliar nutrient concentrations vary largely amongst different species and different individuals of the same species despite similar soil conditions ([24]). The relative importance of site and species, as the factors determining nutrient concentrations in plant biomass, may differ depending on nutrient element and biomass fraction. Comparative studies of several species growing on the same soils allow a better understanding of species nutrient function ([10]).
Different species and clones of poplar are commonly planted as fast-growing species in the world and some parts of plain areas in the north of Iran. Poplar has been planted by the villagers and big companies at different levels in the north of Iran, particularly in Guilan province. Some important species of poplar, such as Populus x canadensis and Populus deltoides (different clones) were commonly used for plantations. On the other hand, Populus caspica (Persian poplar), an endemic and endangered poplar species, existed naturally in plain areas of the Guilan province beside the planted species ([1]).
As these fast-growing tree species provide social, economic and environmental benefits over short rotations, a better understanding of their impacts on nutrient cycling processes and various aspects of soil fertility is essential. The objectives of this study were: (i) to examine the nutrient status in soil and its patterns; (ii) to quantify nutrient return through senescent leaves; and (iii) to evaluate and compare the nutrient retranslocation, in different poplar species and clones.
Materials and Methods
Study area
The study area is located at Safrabasteh poplar research station in Guilan province, Iran (37° 19’ N, 49° 57’ E). The area is on flat and uniform terrain in a plain region with the altitude of -10 m above sea level. Annual mean rainfalls and temperature are 1186 mm and 17.5 °C respectively. The soil of the study area is formed on the alluvial fine textured sediment with silt loam texture, and with neutral to low acidic reaction. The area is dominated by natural forests of native species such as Alnus glutinosa, Pterocarya fraxinifolia, Populus caspica, Gleditschia caspica. Extensive plantations have been established by different poplar species and clones 20 years ago.
Experimental design, tree measurement, soil sampling and analysis
The experimental plantations considered in this study were established in 1993 using a completely randomized block design, including three replications with the following five species/clones (treatments): (i) P.d (Populus deltoides) “Harvard”; (ii) P.d “Lux”; (iii) P.xc (Populus × canadensis) “Triplo”; (iv): P.xc “I-45/51”; and (v) P. caspica. The area of poplar research station was approximately 4 ha and about 3000 m2 were allocated as experimental plantation in this study. After the removal of tree buffer rows, the number of poplar trees was 120 and 24 for all the treatments and per treatment, respectively. In each treatment, there were 3 replications with 8 poplar trees, planted at 4×4 m spacing, assigned to each replication.
Total height, diameter at breast height (DBH) and diameter of crown (by measuring two perpendicular diameters and calculating their mean) were measured for each tree. Three soil samples were randomly taken from 0-20 cm depth of soils under the crown of the tree in each treatment using a core sampler. All of soil samples were air-dried and passed through a 2 mm mesh. Soil organic carbon (OC) was determined by Walkley and Black method and total nitrogen (N) using Kjeldhal method. The available phosphorus (P) was determined according to Olsen methods and exchangeable potassium (K) extracted by using 1 M ammonium acetate at pH 7.0 and analyzed with flame-photometer ([14]).
Leaf collection, leaf nutrients analysis and calculation of retranslocation rate
Live and senescent leaves were collected from six trees in each treatment (two trees per replication). Live leaves were collected in middle of July 2011. The leaves of each tree were collected from the bottom one-third twigs located on the opposite sides of the crown ([30]). The senescent leaves were collected in litter traps (1×1 m with 30 cm height, wooden sides with a nylon net in bottom, located beneath the crown of the same trees previously sampled for live leaves) in November 2011, with the beginning time of leaf fall season ([29]). In order to obtain minimum 3 g of powdered leaf materials, at least 10 leaves were collected for each sample category. All of the collected live and senescent leaves were dried at 70 °C for at least 48 h. The powdered leave materials were analyzed for some important macro elements.
Carbon was determined by loss ignition after heating at 375 °C for 24 h. The total nitrogen was determined with Kjeldahl method, the total phosphorus and potassium was determined after dry ashing and extraction with HCl by colorimetric procedure and flame-photometer, respectively ([14]). Percentage retranslocation was calculated by the following equation ([13], [12] - eqn. 1):
where NRE
is the nutrient resorption efficiency, Nu
live is the nutrient concentration of live leaves (mature leaves) and Nu
dead is the nutrient concentration of senescent leaves (leaf litter).
Statistical analyses
Normality of variables was checked by Kolmogorov-Smirnov test, and Levene’s test was used to examine the quality of the variances. One-way analysis of variance (ANOVA) was used to compare tree quantitative characteristics, soil properties and leaf nutrients data among the experimental treatments. Tukey-HSD test was used to separate the means of dependent variables that were significantly affected by the treatments. For all statistical analyses, SPSS® software (version 17.0) was used.
Results
Quantitative characteristics of trees and soil nutrient status
The results of ANOVA indicated that P.d “Harvard” had the highest total height, DBH and diameter of crown compared to the other species and clones. In contrast, P. caspica showed the lowest performances amongst the treatments, as indicated by average total height, DBH and crown diameter (10.58 m, 13.84 cm and 3.21 m, respectively - Tab. 1).
Tab. 1 - Mean with standard error of height and diameter at breast height (DBH) among the treatments. Different letters indicate significant differences between individual mean values for a particular parameter.
Treatment | Total height (m) |
DBH (cm) |
Diameter of crown (m) |
---|---|---|---|
P. caspica | 10.58 ± 0.34 c | 13.84 ± 1.43 b | 3.21 ± 0.39 c |
P.d “Lux” | 20.58 ± 0.57 a | 20.40 ± 1.54 a | 6.69 ± 0.11 a |
P.d “Harvard” | 22.59 ± 0.62 a | 25.70 ± 2.78 a | 6.71 ± 0.16 a |
P.xc “Triplo” | 16.18 ± 0.41 b | 22.40 ± 1.38 a | 4.12 ± 0.14 b |
P.xc “I-45/51” | 16.16 ± 0.38 b | 20.98 ± 1.80 ab | 4.80 ± 0.17 b |
OC, N and P in the 0-20 cm soil layer differed significantly among poplar clones. The amounts of N and P as the most important nutrient elements and also OC are higher in soil under P.d “Harvard” clone. Soil nutrient status of P. caspica showed the lowest amounts of OC, N and P compared to the other species and clones. Exchangeable potassium did not show any significant differences amongst clones and species (Tab. 2).
Tab. 2 - Mean with standard error of soil nutrient elements among the treatments. Different letters indicate significant differences between individual mean values for a particular parameter.
Treatment | OC (%) |
N (%) |
P (%) |
K (%) |
---|---|---|---|---|
P. caspica | 1.61 ± 0.14b | 0.13 ± 0.01 b | 0.01 ± 0.04 b | 0.01 ± 0.13 a |
P.d “Lux” | 2.41 ± 0.21ab | 0.21 ± 0.01 b | 0.02 ± 0.08 ab | 0.02 ± 0.13 a |
P.d “Harvard” | 2.85 ± 0.31a | 0.24 ± 0.02 a | 0.04 ± 1.01 a | 0.02 ± 0.21 a |
P.xc “Triplo” | 1.78 ± 0.13b | 0.15 ± 0.01 b | 0.01 ± 0.08 b | 0.01 ± 0.13 a |
P.xc “I-45/51” | 1.70 ± 0.27b | 0.14 ± 0.02 b | 0.01 ± 0.04 b | 0.01 ± 0.21 a |
Nutrient concentration in live and senescent leaves
ANOVA results did not show any significant differences between blocks in live and senescent leaves. However, significant differences amongst treatments have been observed. N and P were higher in live leaves of P.d “Harvard” compared to the other clones and species, although N showed significant differences only between P.d “Harvard” and P. caspica (Fig. 1a, b). The amount of K did not show any significant differences for live leaves among the clones and species (Fig. 1c).
Fig. 1 - Concentration of N (a), P (b) and K (c) in live leaves among different treatments (Mean ± SE, n= 6 for each treatment). Different letters above bars indicate significant differences among treatments (P<0.05).
All of the nutrient elements showed significant differences for senescent leaves among the clones and species. OC, N, P, and K return by senescent leaves were higher and lower for P.d “Harvard” and P. caspica, respectively. C/N and C/P ratio for senescent leaves of P. caspica had higher value compared to the other treatments (Tab. 3).
Tab. 3 - Mean of nutrient concentration in senescent leaves with their standard error among the treatments. Different letters indicate significant differences between individual mean values for a particular parameter.
Treatment | OC (%) |
N (%) |
C/N | P (%) |
C/P | K (%) |
---|---|---|---|---|---|---|
P. caspica | 16.23 ± 0.89 | 1.11 ± 0.12 | 15.24 ± 0.12 | 0.01 ± 0.11 | 72.01 ± 1.27 | 0.12 ± 0.19 |
P.d “Lux” | 20.07 ± 1.04 | 1.50 ± 0.17 | 13.47 ± 0.67 | 0.02 ± 0.05 | 45.32 ± 1.63 | 0.22 ± 0.35 |
P.d “Harvard” | 22.34 ± 1.15 | 2.17 ± 0.28 | 11.31 ± 0.34 | 0.03 ± 0.13 | 31.22 ± 1.21 | 0.35 ± 0.73 |
P.xc “Triplo” | 19.18 ± 0.86 | 1.23 ± 0.08 | 14.06 ± 0.51 | 0.01 ± 0.02 | 33.00 ± 1.23 | 0.21 ± 0.24 |
P.xc “I-45/51” | 29.16 ± 1.72 | 1.18 ± 0.17 | 14.21 ± 0.48 | 0.02 ± 0.13 | 42.31 ± 1.08 | 0.13 ± 0.14 |
Total nutrient content was higher in live leaves than senescent leaves. In addition, nutrient concentration in leaves was higher than that in soil.
Nutrient retranslocation
Significant differences in nutrient retranslocation for N and P were observed among the treatments. Among the clones and species, P. caspica had higher retranslocation for all of the nutrient elements. In contrast, nutrient retranslocation in P.d “Harvard” follows opposite trend and the lowest P and N retranslocation were observed for this clone. P retranslocation for all of the clones and species was higher compared to N and K. Retranslocation percentage of the nutrient was in order: P (32.46-39.63%), K (21.14-24.02%) and N (12.09-18.93% - Fig. 2a, b, c).
Fig. 2 - Retranslocation of P (a), K (b) and N (c) among different treatments (Mean ± SE, n= 6 for each treatment). Different letters above bars indicate significant differences among treatments (P<0.05).
Discussion
After about 20 years, different poplar species and clones have had different effects on soil nutrient status. N, P, K and organic carbon exhibited significant differences among the soil under the treatments and their higher and lower amounts were observed in P.d “Harvard” and P. caspica, respectively. As the same aged and adjacent clones and species of poplar were planted and developed under the same site conditions, it seems that the surface soil nutrient differences are due to the nutrient function of each poplar species and clones. Nutrient returns through litter fall under different tree species are important to understand the dynamics of soil fertility, and the rates of forest litter falls and their decomposition contribute to the regulation of nutrient cycling and the maintenance of soil fertility in forest ecosystems ([25]). N, P, K and organic carbon returns by senescent leaves showed significant differences amongst the clones and species. The concentration of all of the nutrient elements in senescent leaves was higher and lower in P.d “Harvard” and P. caspica, respectively. The nutrient returned to the soil through senescent leaves was in the order of N>K>P. Pattern of nutrient concentration in senescent leaves varied according to the species. Similar to our results, Singh et al. ([32]) and Lodhiyal & Lodhiyal ([17]) reported the returned nutrient content by leaf litter in the order of N>K>P in Populus deltoides plantations in moist plain area in the north of India. However, Mahmood et al. ([20], [21]) reported nutrient release from leaf litter of three tree species in Bangladesh follows the order of K>N>P.
The amount of nutrient added to the soil through decomposition of leaf litter depends on climatic conditions, litter quality, and other site conditions such as topographic factors ([23]). In this study, as the climatic and other site conditions for all of the poplar clones and species are the same, the variations in decomposition rate of different tree litters can be resulted from differences among the substrate quality of different tree species. Generally, litter with high lignin and low N concentration has a slower decomposition rate and immobilizes more N than litter with low lignin and high N content. Cornwell et al. ([6]) and Berg et al. ([3]) observed that decomposition depends on the type of humus and on the N and P content of the leaves. In our study, the senescent leaves had higher N, P and K contents in P.d “Harvard” than the other poplar clones and species, and the pattern of C/N was in the order of P. caspica > P.xc “I-45/51” > P.xc “Triplo” > P.d “Lux” > P.d “Harvard”. C/P ratio is also higher in P. caspica compared to the other species and clones. There is a highly significant and negative correlation between C/N and C/P of senescent leaves with N concentration and extractable P in the soil ([2]). Rostamabadi et al. ([29]) reported lower decomposition and release of nutrient from the litter with high C/N and C/P ratio in the plantations in the north of Iran.
Retranslocation percentage of nutrients (NPK) were in the order of P. caspica > P.xc “I-45/51”> P.xc “Triplo” > P.d “Lux” > P.d “Harvard”. Several researches have clearly shown that tree species differ in their retranslocation ([11], [30], [12]). Sharma & Sharma ([31]) suggested that different retranslocation of nutrients depends upon many factors such as, plant growth, species, and stature associated with age and site characteristics. Cuevas & Lugo ([7]) showed that tree species differ in N and P retranslocation and these differences are in response to eco-physiological reactions of each tree to site conditions. In this study, it seems that different response of nutrient retranslocation of poplar species and clones are according to their nutritional requirements and site characteristics. Wright & Westoby ([35]) stated that retranslocation proficiency is a much more accurate indicator of site fertility. According to our results, P. caspica showed the lowest total height, DBH and crown diameter amongst the different species and clones analyzed. The soil under P. caspica also contained poor nutrient supply compared to the other clones and species. Consistent with our results, Liu et al. ([19]) reported that high element retranslocation and low growth rate are the characteristics of plants under element-poor conditions. On the other hand, since the soil under P.d “Harvard” is relatively nutrient-rich, the nutrients were not retranslocated as efficiently as to the other clones and species. Low rate of retranslocation for P.d “Harvard” suggests these trees are less nutrient conservative and the nutrients are being recycled into the soil ([33]).
In our study, the percentage of retranslocation of nutrients (NPK) was in the order P>K>N. Similar to our results, Hashemi et al. ([12]) and Rostamabadi et al. ([29]) reported high P retranslocation in different tree species in northern Iran. In contrast to our results, Lodhiyal & Lodhiyal ([17], [18]) and Rouhi-Moghaddam et al. ([30]) found low P retranslocation in tree layers. The extent to which an element can be retranslocated depends on its physical properties, as well as its importance to the plant’s nutrient requirements ([9]). The agreement between the high P retranslocation and potentially low P content in the soil for P. caspica plantation indicates that P retranslocation can be a strong approach of this species to soil phosphorous availability. So, lower N retranslocation can result in proper balance of nitrogen in the soil.
Conclusion
Understanding the mass and nutrient content of leaves, litter and soil will help to evolve appropriate strategies of nutrient management in plantations. In this study, total height, DBH and diameter of crown, as important tree growth indicators, and OC, N, P, K content of senescent leaves and soil somewhat differed among poplar clones and species in the order: P. caspica < P.xc “Triplo” < P.xc “I-45/51” < P.d “Lux” < P.d “Harvard”. Retranslocation percentage of N and P were in the order of P. caspica > P.xc “I-45/51” > P.xc “Triplo” > P.d “Lux” > P.d “Harvard”, and P retranslocation for all of the clones and species was higher compared to N and K. It could be concluded that the studied poplar species and clones have different nutritional requirements and retranslocation capabilities. It appears that P.d “Harvard” clone showed higher quantitative growth and it could be beneficial for maintaining the soil nutrient status for successive plantings in the north of Iran and other sites similar to the study area.
Acknowledgments
We specially thank to University of Guilan and Poplar Research Station of Safrabasteh. They have guided us through various steps of the preparation of our research.
References
Gscholar
CrossRef | Gscholar
CrossRef | Gscholar
Gscholar
Gscholar
Gscholar
Gscholar
Gscholar
Gscholar
Gscholar
Authors’ Info
Authors’ Affiliation
Neda Ghorbanzadeh
Department of Forestry, Natural Resources Faculty, University of Guilan, Guilan (Iran)
Department of Forestry, Natural Resources Faculty, University of Tehran, Tehran (Iran)
Corresponding author
Paper Info
Citation
Salehi A, Ghorbanzadeh N, Salehi M (2013). Soil nutrient status, nutrient return and retranslocation in poplar species and clones in northern Iran. iForest 6: 336-341. - doi: 10.3832/ifor0976-006
Academic Editor
Roberto Tognetti
Paper history
Received: Feb 18, 2013
Accepted: May 11, 2013
First online: Aug 29, 2013
Publication Date: Dec 02, 2013
Publication Time: 3.67 months
Copyright Information
© SISEF - The Italian Society of Silviculture and Forest Ecology 2013
Open Access
This article is distributed under the terms of the Creative Commons Attribution-Non Commercial 4.0 International (https://creativecommons.org/licenses/by-nc/4.0/), which permits unrestricted use, distribution, and reproduction in any medium, provided you give appropriate credit to the original author(s) and the source, provide a link to the Creative Commons license, and indicate if changes were made.
Web Metrics
Breakdown by View Type
Article Usage
Total Article Views: 29744
(from publication date up to now)
Breakdown by View Type
HTML Page Views: 22818
Abstract Page Views: 1130
PDF Downloads: 4728
Citation/Reference Downloads: 26
XML Downloads: 1042
Web Metrics
Days since publication: 3985
Overall contacts: 29744
Avg. contacts per week: 52.25
Article Citations
Article citations are based on data periodically collected from the Clarivate Web of Science web site
(last update: Nov 2020)
Total number of cites (since 2013): 2
Average cites per year: 0.25
Publication Metrics
by Dimensions ©
Articles citing this article
List of the papers citing this article based on CrossRef Cited-by.
Related Contents
iForest Similar Articles
Research Articles
Retranslocation of foliar nutrients of deciduous tree seedlings in different soil condition under free-air O3 enrichment
vol. 9, pp. 835-841 (online: 17 June 2016)
Research Articles
Growth performance and nitrogen use efficiency of two Populus hybrid clones (P. nigra × P. maximowiczii and P. trichocarpa × P. maximowiczii) in relation to soil depth in a young plantation
vol. 9, pp. 847-854 (online: 22 September 2016)
Research Articles
Links between phenology and ecophysiology in a European beech forest
vol. 8, pp. 438-447 (online: 15 December 2014)
Research Articles
Soil stoichiometry modulates effects of shrub encroachment on soil carbon concentration and stock in a subalpine grassland
vol. 13, pp. 65-72 (online: 07 February 2020)
Research Articles
Kinetic analysis of poplar wood properties by thermal modification in conventional oven
vol. 11, pp. 131-139 (online: 07 February 2018)
Research Articles
Nutrient accumulation and export in teak (Tectona grandis L.f.) plantations of Central America
vol. 8, pp. 33-44 (online: 04 June 2014)
Research Articles
Groundwater uptake of forest and agricultural land covers in regions of recharge and discharge
vol. 9, pp. 696-701 (online: 17 May 2016)
Research Articles
Impacts of Norway spruce (Picea abies L., H. Karst.) stands on soil in continental Croatia
vol. 12, pp. 511-517 (online: 02 December 2019)
Research Articles
Heat treatment of poplar plywood: modifications in physical, mechanical and durability properties
vol. 16, pp. 1-9 (online: 09 January 2023)
Research Articles
Soil fauna communities and microbial activities response to litter and soil properties under degraded and restored forests of Hyrcania
vol. 14, pp. 490-498 (online: 11 November 2021)
iForest Database Search
Search By Author
Search By Keyword
Google Scholar Search
Citing Articles
Search By Author
Search By Keywords
PubMed Search
Search By Author
Search By Keyword