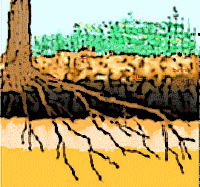
Availability and evaluation of European forest soil monitoring data in the study on the effects of air pollution on forests
iForest - Biogeosciences and Forestry, Volume 4, Issue 5, Pages 205-211 (2011)
doi: https://doi.org/10.3832/ifor0588-004
Published: Nov 03, 2011 - Copyright © 2011 SISEF
Research Articles
Collection/Special Issue: COST Action FP0903 (2010) - Rome (Italy)
Research, monitoring and modelling in the study of climate change and air pollution impacts on forest ecosystems
Guest Editors: E Paoletti, J-P Tuovinen, N Clarke, G Matteucci, R Matyssek, G Wieser, R Fischer, P Cudlin, N Potocic
Abstract
In the study of air pollution effects on forest ecosystems, solid soil data such as cation exchange capacity, base saturation and other exchangeable cation fractions, soil texture, soil moisture, soil weathering rates, C/N ratio and other variables form an important information base for many air pollution impact models. This paper shows some of the possibilities and the limitations of the soil data that European countries collected on the systematic Level I and on the intensive and permanent Level II monitoring plots within the ICP Forests programme. The soil data date from a first inventory in the 1990s and from a second inventory more than 10 years later. Both surveys were conducted following a common manual on sampling and analysis of soil. An example of the changes in pH(CaCl2) and base saturation in the forest floor and mineral soil on more than 2000 plots till a depth of 80 cm between the two surveys is presented. In this period the pH(CaCl2) significantly increased in the very acid forest soils [with pH(CaCl2) below 4.0] but further decreased in forest soils with pH(CaCl2) above 4.0. Following the trend in pH, the base saturation increased in soils with a very low buffering capacity (soils with a base saturation below 20% in the first inventory) and decreased in forest soils with reference base saturation values above 20%. There is both a decrease of soil pH and base saturation in the forest floor of the Arenosols and Podzols. In the Podzols this decreasing trend could not be established in the mineral soil, though this decreasing trend persisted in a number of mineral soil layers of the Arenosols. The only consistent increasing trend of pH and base saturation when stratifying according to the WRB reference soil groups was seen in the forest floor of the Luvisols and Cambisols.
Keywords
Forest soils, Soil acidification, Soil monitoring, ICP Forests
Introduction
One of the factors influencing the condition of European forests is the persistent input of atmospheric pollutants ([17], [27]). In addition to the direct damage to trees, the indirect effects of atmospheric pollutants through the soil are one of the main reasons for the deterioration of the condition of forest ecosystems ([10], [32], [33]). An examination of the soil of the European forests provides basic information on the chemical condition of the soil and the nutrient supply to the trees, as well as the influence of pollutant inputs on the soil condition. There are clear correlations between forest soil chemistry and the deposition of acidity and heavy metals ([10], [29], [13], [19], [20]). Therefore a soil condition assessment provides information on soil related stress factors for forest condition, evidenced by nutrient imbalances or impaired growth.
Since 1985, an annual assessment of the crown condition of forest trees has been carried out within the framework of the International Co-operative Programme on Assessment and Monitoring of Air Pollution Effects on Forests (ICP Forests) of the UN/ECE Convention on Long-range Transboundary Air Pollution and the European Union scheme on the protection of forests against atmospheric pollution. In addition, the condition of the European forest soils is monitored at an approximately time interval of 10 years. A first forest soil condition inventory (using a grid of 16 x 16 km) was executed by 30 countries between 1985 and 1996. The results showed a correlation between the soil chemistry and the atmospheric deposition of nitrogen and acidity. Extremely acid topsoil conditions were found on forest plots located almost exclusively in regions receiving very high atmospheric deposition loads ([34]). A second soil inventory was carried out between 2006 and 2008 within the “BioSoil” demonstration project of the Forest Focus Regulation of the European Commission (DG Environment). One of the aims of the project was to check whether a second survey after approximately 10 years could detect any changes in selected soil parameters. To process the data of the first soil inventory ICP Forest set up the Forest Soil Co-ordinating Centre (FSCC). Further activities of the FSCC are to maintain and improve the soil data from the forest soil condition inventory by updating the Manual on Sampling and Analysis of Soil ([8]) and by controlling the quality of the laboratory analysis by organising interlaboratory comparisons every other year ([4], [5], [6], [7], [9]).
The BioSoil survey completes the soil information on the European level and assesses relevant soil parameters, soil layers and plots not considered in the first survey. On the plots that are reassessed within BioSoil, it provides information on changes in soil chemistry. Within the Framework of the EU LIFE+ FutMon project, FSCC is evaluating the data of this second pan-European forest soil inventory.
This paper aims at providing insight in the possibilities and the limitations of the European forest soil database managed by ICP Forests. In a second part a comparison over time is made of two soil properties important in the assessment of the vulnerability to air pollution: soil reaction (pH in CaCl2) and base saturation.
Material and methods
Monitoring networks
The ICP Forests large-scale Level I forest condition monitoring network provides an annual overview on forest condition based on a 16 x 16 km gridnet covering around 6000 plots in Europe. Surveys include an annual crown condition assessment by the inventory of defoliation, discolouration and damage visible to the trees, foliar chemistry analysis and soil chemistry analysis every 10 years. Complementary to Level I, the intensive monitoring programme on approximately 800 Level II plots provides insight into causes affecting the condition of forest ecosystems and into effects of different stress factors. The major forest types of Europe are represented. Monitoring at these plots includes the assessment of tree growth, crown condition, chemical composition of foliage and soil, and species composition of the ground vegetation on most plots, whereas atmospheric deposition, meteorological variables, soil solution chemistry, ambient air quality, litterfall and phenology are monitored at a subset of Level II plots. In addition, the monitoring of ephiphytic lichens, stand structure and deadwood is being tested on 90 plots.
Soil variables
The forest soil database of ICP Forests consists of 4 main parts (Tab. 1). An overview of the actual content and completeness of the soil database of the Level I soil inventory of the first inventory between 1985 and 1996 is given in Tab. 2. The data availability in the 10-20 cm (not shown here) layer is intermediate between the 0-10 cm (surface layer) and the 20-40 cm. A similar soil survey was mainly conducted in 1995-1996 on 742 Level II plots. Here the soil variables pH(CaCl2), total organic carbon and total organic nitrogen are covered for nearly 100% in the organic layer and the mineral surface layer. Concentrations of exchangeable elements exist for 96% of the plots for the mineral surface layer. Information on the aqua regia extractable macronutrients is available for 90 % of the organic layers. On the other hand, data on physical soil properties (soil texture, amount of coarse fragments, bulk density) is very limited on the Level II plots of the first inventory.
Tab. 1 - The four main components of the forest soils database of ICP Forests.
Monitoring Network |
Level I | Level II | Level I | Level II |
---|---|---|---|---|
Time span of field sampling | 1985-1996 | 1990-2000 | 2004-2008 | 2006-2009 |
N° plots | 5289 | 738 | 4928 | 127 |
N° countries | 30 | 24 | 22 | 17 |
Sampled layers | Forest floor 0-10 cm 10-20 cm |
Forest floor 0-10 cm 10-20 cm 20-40 cm 40-80 cm or recalculated from horizons |
OL, OFH layer, H and peat layers 0-(5)-10 cm 10-20 cm 20-40 cm 40-80 cm |
OL, OFH layer, H and peat layers 0-(5)-10 cm 10-20 cm 20-40 cm 40-80 cm |
Sampled horizons | none | none | main genetic horizons | main genetic horizons |
Methods and measured soil variables/parameters | ICP-Forests Manual ([31]); Commission Regulation EEC no. 926/93 ([2]) |
ICP-Forests Manual ([31]) |
ICP-Forests Manual IIIa (2006); Guidelines for soil description ([16]); Soil geographical database of Eurasia and the Mediterranean: instruction guide 4.0 ([25]) |
Tab. 2 - Number of plots and percentage of total number of plots for which the given variable/parameter for the soil layer under consideration is available in the ICP Forests Level I database of the 1985-1996 survey.
Parameters | Forest floor | 0-10 cm layer | 20-40 cm layer | |||
---|---|---|---|---|---|---|
No. plots | % | No. plots | % | No. plots | % | |
General parameters | ||||||
Altitude class (50 m intervals) | 5289 | 100 | - | - | - | - |
Soil unit (FAO, 1989) | 5013 | 95 | - | - | - | - |
Parent material | 2132 | 40 | - | - | - | - |
Soil parameters at layer level | ||||||
Texture (measured or estimated) | - | - | 3430 | 65 | 510 | 10 |
Bulk density (measured or estimated) | - | - | 2259 | 43 | 371 | 7 |
Coarse fragments (measured or estimated) | - | - | 2448 | 46 | 551 | 10 |
Organic layer mass | 4400 | 83 | - | - | - | - |
pH(CaCl2) | 4262 | 81 | 4686 | 89 | 996 | 19 |
Total N | 4670 | 88 | 4633 | 88 | 996 | 19 |
OC | 4471 | 85 | 4636 | 88 | 995 | 19 |
Carbonate content | 325 | 6 | 1099 | 21 | 553 | 10 |
Exchangeable elements | 1115 | 21 | 2984 | 56 | 987 | 19 |
Macronutrients (P, K, Ca, Mg) | 3173 | 60 | 869 | 16 | 338 | 6 |
Micronutrients and heavy metals (Na, Fe, Al, Mn, Cd, Cr, Cu, Ni, Pb, Zn) |
1503 | 28 | 573 | 11 | 3 | 4 |
In the second soil inventory leading to the BioSoil database, additional variables, samples and sampling depths were included. See Tab. 3 and Tab. 4. The data availability on the 10-20 cm depth layer (not shown here) is again intermediate between the surface (0-10 cm) and the subsurface layers (20-40 cm and 40-80 cm).
Tab. 3 - Number of plots and percentage of total number of plots for which the given variable/parameters for the organic layer is available in the BioSoil Level I database of the 2006-2008 survey.
Parameters | OFH - layer | H-layers (peat) | ||
---|---|---|---|---|
N plots | % | N plots | % | |
pH(CaCl2) | 3546 | 72 | 241 | 95 |
pH(H2O) | 2848 | 58 | 231 | 91 |
Total N | 3544 | 72 | 241 | 95 |
OC | 3544 | 72 | 241 | 95 |
Organic layer mass | 3476 | 71 | 144 | 56 |
Carbonate content | - | 0 | 15 | 6 |
Exchangeable elements | 2784 | 56 | 201 | 79 |
Macro - and micronutrients, heavy metals | 3934 | 80 | 241 | 95 |
Total elements (Al, Ca, Fe, K, Mg, Mn, Na) | 133 | 3 | - | - |
Acid oxalate extractable Fe and Al | 1321 | 27 | 110 | 43 |
Tab. 4 - Number of plots and percentage of total number of plots for which the given variable/parameters for the mineral surface and subsurface layer is available in the BioSoil Level I database of the 2006-2008 survey.
Parameters | 0 - 10 cm | 20 - 40 cm | 40 - 80 cm | |||
---|---|---|---|---|---|---|
No. plots | % | No. plots | % | No. plots | % | |
Texture class (and % clay, silt and sand) | 3722 | 80 | 3228 | 69 | 2958 | 63 |
Measured or estimated bulk density of the fine earth | 4180 | 89 | 3228 | 69 | 2926 | 63 |
Coarse fragments (vol % or mass %) | 3549 | 76 | 2614 | 56 | 2268 | 49 |
pH(CaCl2) | 4350 | 93 | 3320 | 71 | 3036 | 65 |
pH(H2O) | 3586 | 77 | 3195 | 68 | 2910 | 62 |
Total Organic Carbon | 4345 | 93 | 3320 | 71 | 3035 | 65 |
Total N | 4345 | 93 | 2774 | 59 | 2534 | 54 |
Carbonates | 1130 | 24 | 902 | 19 | 873 | 19 |
Exchangeable acidity, free H, Fe, Al, Mn, Ca, K, Mg, Na | 3956 | 85 | 3245 | 69 | 2927 | 63 |
Macronutrients (P, Ca, K, Mg, Mn) | 3977 | 85 | 2593 | 55 | 2373 | 51 |
Micronutrients and heavy metals (Cu, Pb, Cd, Zn, Al, Fe, Cr, Ni, S, Na) | 4046 | 87 | 1409 | 30 | 1366 | 29 |
Acid oxalate extractable Al and Fe | 2473 | 53 | 2392 | 51 | 2179 | 47 |
Total Al, Ca, Fe, K, Mg, Mn, Na | 543 | 12 | 496 | 11 | 393 | 8 |
Quality assurance and quality control
In the period between the first and the second forest soil inventory the FSCC and the Expert Panel on Soil and Soil Solution of ICP Forests made major efforts to improve the quality of the soil data through the regular update of the Manual on Sampling and Analysis of Soil ([8]), by the organisation of interlaboratory comparisons ([4], [5], [6], [7], [9]) and by the distribution of a forest soil reference material. Important improvements in the BioSoil database compared to the database based on the first inventory are:
The application of harmonised methods in the field:
- All countries use the same fixed depth intervals [0-(5)-10-20-40-80 cm] for sampling of the composite samples. Sampling according to horizons is restricted to the sampling of the profile pit mainly for soil classification purposes.
- The organic layer is not sampled as a whole but according to the subhorizons OL, OF, OH, Hf, Hfs, Hs) and peat layers are designated with a separate code.
- A minimum number of subsamples are to be included in the composite sample.
- Minimum requirements have been set to the sampling design concerning the spatial distribution of the sampling points across the monitoring plot.
The laboratory methods have been refined, for example:
- Particle size distribution is to be conducted by the pipette method ([23]) and the texture class is derived using the USDA texture triangle ([15]);
- The distinction between “total” (or extractable by aqua regia) and “total total” (complete destruction of the sample) analysis have been made following ISO standards.
All laboratories reporting soil data to the database had to use the reference methods:
- The reference methods follow the ISO standards whenever applicable.
- The laboratory performance is followed up by interlaboratory comparisons every other year.
- During the BioSoil survey, the laboratories could use the FSCC soil reference material for internal quality control.
Forest floor and soil classification:
- Harmonised definitions of humus forms and the application of the general level of the European Humus Classification System (advised by [36]).
- Supporting profile description and laboratory analyses are mandatory according to FAO ([16]) based guidelines.
- The classification following the World Reference Base for Soil Resources 2006 ([22]) is mandatory.
Geographically there is a substantial improvement in the overlay with the tree vitality inventory on the Level I plots. In the first inventory the forest soil condition was often conducted on (a subset of) national forest inventory plots while in the BioSoil survey, the systematic 16 x 16 km grid has been better respected and provides a representative sample of the forest soils in Europe.
Change in pH(CaCl2) and base saturation on the Level I plots
The first and the second pan-European forest soil surveys have more than 2000 Level I plots in common where the pH(CaCl2) in the upper 10 cm of the mineral soil is available. Although there has been a minor change in the reference method, where previously the 1:5 ratio was measured on weight basis where currently a volumetric basis is used, it may be assumed that methodological differences are minor for this soil variable. This assumption is further supported by the small coefficients of variation for pH(CaCl2) in the FSCC interlaboratory comparisons which have been conducted at regular time intervals between both surveys.
To gain a better understanding in the changes of the pH(CaCl2), the variable has been split into five classes. The same pH(CaCl2) class limits as applied in the Forest Soil Condition Report ([34]) have been used (pH ≤ 3.2, 3.2 < pH ≤ 4.0, 4.0 < pH ≤ 5.0, 5.0 < pH ≤ 6.0 and pH > 6.0) to allow comparability with the first inventory.
A second important forest soil property is the base saturation (BS). The changes in % BS between the first and the second inventory are compared. The BS has been calculated as the sum of the basic exchangeable cations (Ca , Mg, K and Na) to the effective cation exchange capacity of the soil (by extraction in a unbuffered BaCl2 solution). Similar to the pH(CaCl2) the BS values were split into five classes following the limits used in the evaluation of the first inventory: BS ≤ 10%, 10% < BS ≤ 20%, 20 < BS ≤ 50%, 50 < BS ≤ 95% and BS > 95%. As the BS in the forest floor was never below 20% or above 95%, different classes limits were applied for this forest floor: BS ≤ 50%, 50 < BS ≤ 60%, 60 < BS ≤ 70%, 70 < BS ≤ 80% and BS > 80%.
A second stratification was conducted following the Reference Soil Groups of the WRB Soil Classification ([21], [22]) both for the pH(CaCl2) and the BS values in the forest floor and the mineral soil layer 0-10, 10-20, and 20-40 cm.
Statistics were calculated using the TIBCO SQPUGJSF S+ 8.1 GPS WJOEPXC (2008). Bootstrapping was used as a resampling technique to obtain confidence intervals (C.I.s) for the plotwise paired mean difference in soil pH(CaCl2) between the two surveys. In the bootstrap, B new samples, each of the same size as the observed data, are drawn with replacement from the observed data. For more information on the bootstrapping technique, see Johnson et al. ([24]). The applied number of resamples (B) was 5000. Confidence intervals were calculated based on bias-corrected and accelerated (Bca) percentiles at 2.5 and 97.5%, respectively.
Results and discussion
Mean paired differences of pH(CaCl2) and BS were computed for each Level I plot as the difference between the values of the second value minus the value of the first survey where negative values indicate a decrease of the variable relative to the first survey. Tab. 5 shows an overall significant decrease of 0.03 pH units in the 0-10 cm mineral soil layer on 2204 plots. With increasing soil depth, the data availability decreases, though an increasing difference till 0.06 pH units is seen in the deeper soil layers.
Tab. 5 - The plotwise paired mean difference of pH(CaCl2) in the forest floor and the four fixed depth mineral layers (0-10 cm, 10-20 cm, 20-40 cm and 40-80 cm) according to 5 pH classes for common plots in the Level I surveys of 1985-1996 and 2004-2008. The last 2 columns show the 95 % confidence interval (95% C.I.) after bootstrapping the mean difference and an indication if this mean difference is significant different from zero (no change). (**): significant at 95% confidence limits; (ns): not significant.
Layer | pH Class |
pH (CaCl2) range | No. observations |
Mean difference | 95 % C.I. |
Prob. |
---|---|---|---|---|---|---|
Forest floor (OF &OH layer) |
1 | ≤ 3.2 | 292 | 0.18 | [0.15;0.22] | ** |
2 | 3.2 - 4 | 498 | -0.01 | [-0.04;0.03] | ns | |
3 | 4 - 5 | 324 | 0.01 | [-0.04;0.07] | ns | |
4 | 5 - 6 | 186 | -0.04 | [-0.13;0.05] | ns | |
5 | > 6 | 144 | -0.20 | [-0.3;-0.11] | ** | |
- | whole | 1444 | 0.01 | [-0.01;0.04] | ns | |
Mineral topsoil (0 - 10 cm) |
1 | ≤ 3.2 | 119 | 0.27 | [0.21;0.33] | ** |
2 | 3.2 - 4 | 829 | 0.06 | [0.04;0.08] | ** | |
3 | 4 - 5 | 583 | -0.04 | [-0.08;0.001] | ** | |
4 | 5 - 6 | 199 | -0.19 | [-0.27;-0.1] | ** | |
5 | > 6 | 452 | -0.19 | [-0.24;-0.15] | ** | |
- | whole | 2204 | -0.03 | [-0.05;-0.01] | ** | |
Mineral soil (10 - 20 cm) |
1 | ≤ 3.2 | 29 | 0.25 | [0.15;0.4] | ** |
2 | 3.2 - 4 | 564 | 0.09 | [0.07;0.12] | ** | |
3 | 4 - 5 | 934 | -0.03 | [-0.06;-0.01] | ** | |
4 | 5 - 6 | 149 | -0.22 | [-0.32;-0.11] | ** | |
5 | > 6 | 434 | -0.18 | [-0.23;-0.14 | ** | |
- | whole | 2110 | -0.04 | [-0.06; -0.02] | ** | |
Mineral soil (20 - 40 cm) |
1 | ≤ 3.2 | 7 | 0.65 | [0.46;0.87] | ** |
2 | 3.2 - 4 | 171 | 0.20 | [0.13;0.3] | ** | |
3 | 4 - 5 | 311 | -0.08 | [-0.11;-0.03] | ** | |
4 | 5 - 6 | 33 | -0.03 | [-0.27;0.29] | ns | |
5 | > 6 | 146 | -0.34 | [-0.47;-0.25] | ** | |
- | whole | 668 | -0.06 | [-0.1;-0.01] | ** | |
Mineral soil (40 - 80 cm) |
2 | ≤ 4 | 74 | 0.14 | [0.07;0.32] | ** |
3 | 4 - 5 | 240 | -0.04 | [-0.08;0.03 | ns | |
4 | 5 - 6 | 32 | -0.11 | [-0.34;0.2] | ns | |
5 | > 6 | 87 | -0.27 | [-0.42;-0.14] | ** | |
- | whole | 433 | -0.06 | [-0.1;-0.003] | ** |
The stratification according to five pH classes allows more differentiation within this general picture. A statistical significant increase in pH (CaCl2) between the second and the first soil inventory of 0.27 pH units is seen in the upper mineral soil layer in soils with a reference pH (first survey) lower than or equal to 3.2. In the pH class 3.2 to 4.0, this significant increase comes down to 0.05 pH units. In the higher pH classes, an opposite trend is seen, namely a decrease between 0.04 and 0.19 pH units. Similar trends could be found in the deeper mineral soil layers. Note that the available data for the 40-80 cm in the first inventory was limited to only 4 countries (433 plots) of which two countries only sampled up to 60 cm depth. In the organic layers statistically significant differences were seen in pH class 1 (increase) and pH class 5 (decrease).
Tab. 6 shows that the BS in the mineral soil significantly increases with 4 to 6% in all mineral soil layers up to 40 cm depth when the reference BS (first survey) was below 20%. When the BS in the first survey was above 20%, a statistically significant decrease is observed between 1 and 15% in most of the mineral soil layers. In the forest floor, where the level of BS is higher compared to the mineral soil, a statistically positive trend (increase in BS of 5%) is seen when BS was below 50% and a statistically negative trend (decrease between 5 and 9%) when the BS was above 60%.
Tab. 6 - The plotwise paired mean difference in % base saturation (BS) in the forest floor and the four fixed depth mineral layers (0-10 cm, 10-20 cm, 20-40 cm and 40-80 cm) according to 5 classes for common plots in the Level I surveys of 1985-1996 and 2004-2008. The last 2 columns show the 95 % confidence interval after bootstrapping the mean difference and an indication if this mean difference is significant different from zero (no change). (**): significant at 95% confidence limits; (*): significant at 90% confidence limits; (a): when statistically significant at the 90 % but not at the 95% significance level; the 90% confidence interval is given; (ns): non significant.
Layer | BS class |
BS range (%) | No. observations |
Mean difference | 95 % C.I. a |
Prob. |
---|---|---|---|---|---|---|
Forest Floor (OF & OH layer) |
1 | ≤ 50 | 67 | 5.0 | [2.5; 7.5] | ** |
2 | 50 - 60 | 97 | -1.6 | [-3.5; 0.3] | ns | |
3 | 60 - 70 | 101 | -4.7 | [-6.3; -2.9] | ** | |
4 | 70 - 80 | 82 | -7.6 | [-9.5; -5.9] | ** | |
5 | > 80 | 62 | -8.7 | [-10.8; -6.7] | ** | |
- | whole | 409 | -3.5 | [-4.6; -2.5] | ** | |
Mineral topsoil (0 - 10 cm) |
1 | ≤ 10 | 165 | 5.8 | [4.3; 8.3] | ** |
2 | 10 - 20 | 301 | 5.2 | [3.7; 7] | ** | |
3 | 20 - 50 | 413 | -1.8 | [-3.2; -0.2] | * | |
4 | 50 - 95 | 344 | -5.5 | [-7.5; -3.6] | ** | |
5 | > 95 | 336 | -2.9 | [-4.1; -2.1] | ** | |
- | whole | 1559 | -0.7 | [-1.4; 0.1] | ns | |
Mineral soil (10 - 20 cm) |
1 | ≤ 10 | 226 | 5.7 | [4.2; 8] | ** |
2 | 10 - 20 | 262 | 4.7 | [3.1; 6.6] | ** | |
3 | 20 - 50 | 428 | -1.7 | [-3.5; 0.1] | ns | |
4 | 50 - 95 | 303 | -8.7 | [-11; -6.2] | ** | |
5 | > 95 | 280 | -3.7 | [-5.5; -2.5] | ** | |
- | whole | 1499 | -1.3 | [-2.2; -0.3] | ** | |
Mineral soil (20 - 40 cm) |
1 | ≤ 10 | 126 | 5.7 | [3.5; 9.7] | ** |
2 | 10 - 20 | 100 | 3.6 | [0.5; 7.7] | ** | |
3 | 20 - 50 | 133 | -8.0 | [-10.9; -4.7] | ** | |
4 | 50 - 95 | 122 | -14.5 | [-19; -9.9] | ** | |
5 | > 95 | 177 | -3.3 | [-6.1; -1.9] | ** | |
- | whole | 658 | -3.6 | [-5.1; -2] | ** | |
Mineral soil (40 - 80 cm) |
1 | ≤ 10 | 21 | 4.0 | [1; 11.3] | ** |
2 | 10 - 20 | 27 | 4.6 | [-1.4; 16.9] | ns | |
3 | 20 - 50 | 31 | -7.9 | [-13.6; -1.1] | ** | |
4 | 50 - 95 | 36 | -7.2 | [-15.3; -0.7] | * | |
5 | > 95 | 43 | -0.8 | [-2.9; -0.1] | ** | |
- | whole | 158 | -0.8 | [-5.2; 0.8] | ns |
Tab. 7 shows the results of the stratification of the changes in pH(CaCl2) and % BS according to the nine most frequently described WRB Reference Soil Groups (RSG) on the Level I plots. The information on the 40-80 cm layer is not shown as the data availability is rather limited. The trend when stratifying according the RSGs is less straightforward compared to the trends seen in the stratification according to the pH and BS classes. There is a decrease of soil pH and BS in the forest floor of the Arenosols and Podzols. In the Podzols this decreasing trend did not persist in the mineral soil though this decreasing trend continued in a number of mineral soil layers of the Arenosols. The only consistent increasing trend of pH and BS was seen in the forest floor of the Luvisols and Cambisols.
Tab. 7 - The plotwise paired mean difference in pH(CaCl2) and % base saturation (BS) in the forest floor and three fixed mineral layers (0-10 cm, 10-20 cm and 20-40 cm) according to the most frequently found WRB Reference Soil Groups (RSG) on common plots in the Level I surveys of 1985-1996 and 2004-2008. (**): significant at 95% significance level; (*): significant at 90% significance level; (a): The 90% confidence interval is given when it was significant at the 90% thought not significant at the 95% significance level; (ns): non significant.
Layer |
WRB RSG |
pH (CaCl2) | Prob. | % base saturation | Prob. | ||||
---|---|---|---|---|---|---|---|---|---|
No. plots |
Mean difference |
95% C.I. a | No. plots |
Mean difference |
95% C.I. a | ||||
Forest floor | Cambisols | 335 | 0.09 | [0.05; 0.14] | ** | 57 | -1.7 | [-4.9; 1.1] | - |
Regosols | 302 | 0.02 | [-0.04; 0.06] | - | 153 | -3.9 | [-5.5; -2.3] | ** | |
Podzols | 196 | -0.05 | [-0.10; -0.001] | ** | 121 | -4.0 | [-5.6; -2.4] | ** | |
Arenosols | 135 | -0.09 | [-0.16; -0.01] | ** | 44 | -6.2 | [-9.4; -3.7] | ** | |
Luvisols | 92 | 0.10 | [0.01; 0.19] | * | 2 | - | - | - | |
Leptosols | 135 | -0.06 | [-0.17; 0.03] | - | 14 | -6.9 | [-9.6; -4.2] | ** | |
Umbrisols | 55 | 0.02 | [-0.08; 0.17] | - | 1 | - | - | - | |
Stagnosols | 30 | 0.05 | [-0.05; 0.15] | - | 16 | 4.7 | [-3.2; 11.4] | - | |
Gleysols | 21 | 0.01 | [-0.26; 0.32] | - | 0 | - | - | - | |
0-10 cm | Cambisols | 645 | -0.02 | [-0.06; 0.02] | - | 498 | 0.7 | [-0.8; 2.2] | - |
Regosols | 368 | -0.04 | [-0.08; -0.008] | ** | 275 | -0.3 | [-4.7; -0.9] | ** | |
Podzols | 252 | -0.01 | [-0.06; 0.03] | - | 181 | -0.8 | [-2.4; 0.9] | - | |
Arenosols | 182 | -0.08 | [-0.13; -0.02] | ** | 145 | -3.7 | [-6.4; -0.8] | ** | |
Luvisols | 146 | 0.01 | [-0.08; 0.09] | - | 104 | 3.9 | [0.8; 7.8] | ** | |
Leptosols | 213 | -0.06 | [-0.11; -0.002] | * | 89 | 1.0 | [-0.4; 3.6] | - | |
Umbrisols | 82 | -0.03 | [-0.15; 0.07] | - | 60 | -4.4 | [-8.8; 1.4] | - | |
Stagnosols | 57 | -0.01 | [-0.10; 0.08] | - | 53 | -4.2 | [-8.7; -0.1] | * | |
Gleysols | 48 | 0.04 | [-0.12; 0.19] | - | 33 | 5.6 | [1.3; 11.5] | ** | |
10-20 cm | Cambisols | 610 | -0.06 | [-0.1; -0.02] | ** | 472 | -1.2 | [-2.8; 0.4] | - |
Regosols | 361 | -0.03 | [-0.06; -0.002] | * | 272 | -1.2 | [-3.6; 1] | - | |
Podzols | 232 | 0.00 | [-0.04; 0.03] | - | 181 | -1.3 | [-3.2; 0.7] | - | |
Arenosols | 166 | -0.05 | [-0.09; -0.006] | * | 144 | -1.4 | [-4.7; 1.7] | - | |
Luvisols | 140 | -0.01 | [-0.10; 0.08] | - | 105 | 3.6 | [0.1; 7.7] | * | |
Leptosols | 177 | -0.02 | [-0.08; 0.05] | - | 70 | 1.5 | [-0.6; 4.3] | - | |
Umbrisols | 78 | -0.04 | [-0.10; 0.05] | - | 58 | -10.6 | [-16.4; -4.1] | ** | |
Stagnosols | 53 | 0.01 | [-0.07; 0.15] | - | 52 | -4.0 | [-7.6; -0.4] | * | |
Gleysols | 46 | -0.02 | [-0.2; 0.16] | - | 32 | 0.2 | [-5.2; 7.0] | - | |
20-40 cm | Cambisols | 290 | -0.04 | [-0.10; 0.03] | - | 288 | -0.7 | [-2.7; 1.4] | - |
Regosols | 64 | -0.10 | [-0.24; -0.009] | ** | 61 | -8.7 | [-15.3; -3.5] | ** | |
Podzols | 37 | 0.10 | [-0.006; 0.22] | - | 36 | -1.1 | [-7.4; 5.8] | - | |
Arenosols | 64 | 0.01 | [-0.04; 0.07] | - | 64 | -8.7 | [-12.5; -5.2] | ** | |
Luvisols | 24 | 0.00 | [-0.30; 0.36] | - | 24 | 0.1 | [-12.1; 13.5] | - | |
Leptosols | 24 | -0.06 | [-0.31; 0.10] | - | 2 | - | - | - | |
Umbrisols | 34 | -0.11 | [-0.22; 0.03] | - | 34 | -6.9 | [-14.7; 2.3] | - | |
Stagnosols | 34 | -0.06 | [-0.29; 0.08] | - | 34 | -11.2 | [-18.8; -6.1] | ** | |
Gleysols | 21 | -0.23 | [-0.50; -0.005] | ** | 20 | -1.9 | [-12.3; 6.9] | - |
As pH(CaCl2) and BS are strongly correlated in forest soils ([1], [34]), the analyses of the trend for both properties seem to show a recovery after acidification in the most acid forest soils [with pH(CaCl2) ≤ 4.0 or BS ≤ 20%]. These acid and/or strongly leached soils are mainly found in north and northwest Europe. These findings are supported by the study of Matschonat & Falkengren-Grerup ([28]) who observed in southern Sweden between 1988 and 1996 increase in the exchange saturation and pH only in those soils with small cation exchange capacity and low buffering capacity. Also Vanguelova et al. ([35]) found a recovery of soil pH between 1995 and 2006 on sites with very low buffering capacities. On the other hand a further acidification between roughly 1994 and 2006 is observed on the forest soils with pH(CaCl2) above 4.0 or a base saturation above 20% in the mineral soil. One possible explanation for the observed changes is the decreased atmospheric deposition. Soils with relatively high pH, base saturation and cation exchange capacity are better represented in the southern parts of Europe. While atmospheric deposition has decreased whole over Europe over the past three decades ([12]), also the deposition of base cations decreased ([18], [26]). This decrease might have a different influence on base saturation in a variety of soil types in different part of Europe. Draaijers et al. ([11]) showed that soils in eastern and southern Europe mainly rely on atmospheric deposition for the supply of base cations while in Scandinavia weathering is the dominant supplier of basic cations.
These trends of recovery and decrease of soil pH and BS are similar in the 0-10 cm and 10-20 cm layers while the difference in the lowest and highest pH classes and highest BS classes are more explicit in the 20-40 cm layer. Based on the current knowledge of forest soil acidification and the related recovery processes, these trends are hard to explain. Sverdrup et al. ([30]) postulated rather a poorer recovery with increasing soil depth based on the theory that due to differences in properties of individual soil horizons, different parts of the soil profile acidify and recover at different rates. In the acidification process, there could be a significant delay from the topsoil, which is first affected by acid deposition, to the bottom of the soil profile. During acidification, hydrogen and Al+ ions mobilized in the soil solution may exchange with the base cations on a cation exchangeable complex and delay the decrease in pH. During recovery, the reverse process could occur, and while the upper layers recover, simultaneously the bottom layers may still acidify. This phenomenon will be further investigated based on the gradients in pH and BS within each forest soil profile.
Further investigations on possible relationships between the established changes in pH and base saturation with other soil variables such as exchange capacity of the soil, soil texture, organic carbon content, nitrogen and anthropogenic pollutants (such as Pb) will be conducted. These correlation studies will lead to a better understanding of the detected changes.
The classification of the Level I plots according to the World Reference Base for Soil Resources ([21], [22]) provides an important added value of the ICP Forests database as this harmonised information cannot be deduced from soil maps at the European scale (such as the Soil Atlas of Europe by the European Soil Bureau Network - [14]) as these maps are mainly based on data from agricultural land whereas the situation in forests might be completely different.
Conclusions
The ICP Forests soil database provides a very valuable basis for the monitoring of forest soil data. An often overlooked but very important benefit of the ICP Forests databases in comparison with other Pan-European forest databases, is that the data have been collected by applying harmonised methods both in the field and in the laboratory and that the laboratory performance is guarded by regular interlaboratory comparisons. The current Level I database contains 2421 plots included in two forest soil inventories with an average time interval of 12 years enabling statistical powerful comparisons. Notwithstanding the limitiation that a number of parameters and laboratory methods have changed over time, the variables pH(CaCl2), organic carbon, total nitrogen, exchangeable elements and aqua regia extractable elements in the mineral and organic layer offer valuable monitoring information on the status and trends of European forest soil condition. Over a period of approximately 12 years (1994-2006) statistically significant changes in soil pH and base saturation could be established. It however asks for more in depth investigations to fully understand these changes.
Acknowledgements
The authors wish to acknowledge the ICP Forests National Focal Centres of Austria, Belgium, Czech Republic, Cyprus, Denmark, Estonia, Finland, France, Germany, Greece, Hungary, Ireland, Italy, Latvia, Lithuania, Poland, Portugal, Serbia, Slovenia, Slovak Republic, Spain and the United Kingdom for making the BioSoil data available to the Forest Soil Coordinating Centre for analysis and evaluation at the European level within the framework of the FutMon project under the LIFE+ Regulation (EC) No. 614/2007 of the European Parliament and of the Council. Financial support for the data collection of both survey was granted by the European Commission through Commission Regulation (EEC) No. 926/93 ([2]) and the European Commission Forest Focus Regulation (EC) No. 2152/2003 ([3]).
References
Gscholar
Gscholar
Gscholar
Gscholar
Gscholar
Gscholar
Gscholar
Gscholar
Gscholar
Gscholar
Gscholar
Gscholar
Gscholar
Gscholar
Gscholar
Gscholar
Gscholar
CrossRef | Gscholar
Authors’ Info
Authors’ Affiliation
Corresponding author
Paper Info
Citation
Cools N, De Vos B (2011). Availability and evaluation of European forest soil monitoring data in the study on the effects of air pollution on forests. iForest 4: 205-211. - doi: 10.3832/ifor0588-004
Paper history
Received: Nov 30, 2010
Accepted: May 15, 2011
First online: Nov 03, 2011
Publication Date: Nov 03, 2011
Publication Time: 5.73 months
Copyright Information
© SISEF - The Italian Society of Silviculture and Forest Ecology 2011
Open Access
This article is distributed under the terms of the Creative Commons Attribution-Non Commercial 4.0 International (https://creativecommons.org/licenses/by-nc/4.0/), which permits unrestricted use, distribution, and reproduction in any medium, provided you give appropriate credit to the original author(s) and the source, provide a link to the Creative Commons license, and indicate if changes were made.
Web Metrics
Breakdown by View Type
Article Usage
Total Article Views: 26608
(from publication date up to now)
Breakdown by View Type
HTML Page Views: 20180
Abstract Page Views: 1312
PDF Downloads: 3737
Citation/Reference Downloads: 32
XML Downloads: 1347
Web Metrics
Days since publication: 4650
Overall contacts: 26608
Avg. contacts per week: 40.06
Article Citations
Article citations are based on data periodically collected from the Clarivate Web of Science web site
(last update: Nov 2020)
Total number of cites (since 2011): 19
Average cites per year: 1.90
Publication Metrics
by Dimensions ©
Articles citing this article
List of the papers citing this article based on CrossRef Cited-by.
Related Contents
iForest Similar Articles
Research Articles
Soil chemical and physical status in semideciduous Atlantic Forest fragments affected by atmospheric deposition in central-eastern São Paulo State, Brazil
vol. 8, pp. 798-808 (online: 22 April 2015)
Research Articles
Soil fauna communities and microbial activities response to litter and soil properties under degraded and restored forests of Hyrcania
vol. 14, pp. 490-498 (online: 11 November 2021)
Research Articles
Soil stoichiometry modulates effects of shrub encroachment on soil carbon concentration and stock in a subalpine grassland
vol. 13, pp. 65-72 (online: 07 February 2020)
Research Articles
The manipulation of aboveground litter input affects soil CO2 efflux in a subtropical liquidambar forest in China
vol. 12, pp. 181-186 (online: 10 April 2019)
Research Articles
Wood-soil interactions in soil bioengineering slope stabilization works
vol. 2, pp. 187-191 (online: 15 October 2009)
Research Articles
Soil respiration along an altitudinal gradient in a subalpine secondary forest in China
vol. 8, pp. 526-532 (online: 01 December 2014)
Research Articles
Impact of deforestation on the soil physical and chemical attributes, and humic fraction of organic matter in dry environments in Brazil
vol. 15, pp. 465-475 (online: 18 November 2022)
Research Articles
Comparison of soil CO2 emissions between short-rotation coppice poplar stands and arable lands
vol. 11, pp. 199-205 (online: 01 March 2018)
Research Articles
Spatial heterogeneity of soil respiration in a seasonal rainforest with complex terrain
vol. 6, pp. 65-72 (online: 07 February 2013)
Research Articles
Seasonal dynamics of soil respiration and nitrification in three subtropical plantations in southern China
vol. 9, pp. 813-821 (online: 29 May 2016)
iForest Database Search
Search By Author
Search By Keyword
Google Scholar Search
Citing Articles
Search By Author
Search By Keywords
PubMed Search
Search By Author
Search By Keyword