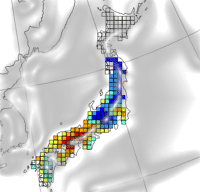
A comparison between stomatal ozone uptake and AOT40 of deciduous trees in Japan
iForest - Biogeosciences and Forestry, Volume 4, Issue 3, Pages 128-135 (2011)
doi: https://doi.org/10.3832/ifor0573-004
Published: Jun 01, 2011 - Copyright © 2011 SISEF
Research Articles
Abstract
A comparison of the maps of stomatal ozone uptake (AFst0) and concentrations exceeding 40 ppb (AOT40) for dominant temperate deciduous tree species (Quercus serrata, Fagus crenata, Betula ermanii) was conducted in Japan. Estimations of stomatal ozone uptake were accomplished using estimated ozone concentration, climate data, and vegetation data. Key parameters such as stomatal conductance parameters for each species were collected from scientific literature in Japan. Stomatal closure induced by vapour pressure deficit affected the AFst0 values in warmer part of Japan. For this reason, the areas with high AOT40 did not always correspond to the areas with high AFst0. The result showed that ozone risk assessment using AOT40 is VPD-constrained in central Japan, which implies an overestimation of risk compared to AFst0. While in Europe AOT40 is higher where water stress is recurrent, AOT40 peaked in the cool and humid climate region of central-eastern Japan where also stomatal ozone uptake reached maximum values.
Keywords
Deciduous forest trees, Ozone uptake modeling, Stomatal conductance, Japan, Ozone
Introduction
Phytotoxic nature of ozone has been well known for decades (e.g., [16], [17]). Surface ozone concentrations are increasing in East Asia because of rapid increases in emissions of the main critical ozone precursors, Nitrogen oxides and volatile organic compounds ([18]). Ohara & Sakata ([21]) reported that the annual average concentration of photochemical oxidant, mainly ozone, increased with high rate (0.33 ppb year-1) from 1985 to 1999 in Japan. Therefore, assessments of ozone impacts on plants have become very significant in Japan.
In Japan, effects of ozone on the growth of forest tree species have been investigated by using AOT40, which represents the cumulative exposure above a hourly threshold concentration of 40 ppb during daylight hours (e.g., [9], [13]). Kohno et al. ([13]) suggested that 8-15 ppm·h AOT40 for sensitive species (e.g., Fagus crenata), and 15-30 ppm·h AOT40 for moderately sensitive species (e.g., Quercus serrata) from April to September as critical level for forest species, are able to induce a 10% growth reduction. Critical levels for forest trees are set at 5 ppm·h AOT40 corresponding to a 5 % growth reduction in Europe ([35]). An assessment approach using AOT40 has the advantage of being simple because only atmospheric ozone concentration data are needed. However, ozone damage to plants depends not only on atmospheric ozone concentrations but also on stomatal ozone uptake into leaves. Several studies suggested that stomatal ozone uptake was closely related to ozone damages (e.g., [24], [28]). For this reason, a stomatal flux-based approach using stomatal ozone uptake is expected to provide better assessments of ozone damage to plants in Europe ([3]). Critical levels for ozone risk using the stomatal flux-based indices were set for potato and wheat, and provisionally for sensitive forest species (beech, birch - [35]). Karlsson et al. ([11]) reported that the stomatal flux-based indices were superior to AOT40 for ozone-sensitive species, based on a reanalysis of published ozone exposure-response data.
Very little has been done to develop the stomatal flux-based approach for assessing ozone damage to plants in Japan. The assessment approach using AOT40 was suggested to be appropriate for Japan because of much precipitation and limited water stress in Japan ([36]). However, this assumption has not been verified yet. In this study, we developed a model of cumulative stomatal ozone uptake (AFst0) and assess the spatial distribution of AFst0 compared to AOT40 for deciduous forest tree species in Japan.
Materials and methods
Vegetation data
Deciduous forest tree species were classified into three forest types within two climatic zones (cool-temperate and warm-temperate zones) in Japan ([20]). Cool-temperate zone and warm-temperate zone are mainly in northern Japan and western Japan, respectively. Regarding the forest types, cool-temperate mixed broadleaf/conifer forest and cool-temperate deciduous forest are distributed in the cool-temperate zone. Cool-temperate deciduous forest is just in central-eastern Japan, with a humid climate throughout the year and heavy snow in winter. In the cool-temperate deciduous forest, beech (Fagus crenata) is the dominant species. The cool-temperate mixed forest is dominated by deciduous oak (e.g., Quercus crispula) and birch (Betula ermanii). In the warm-temperate zone, the forest type is classified as warm-temperate deciduous forest, and is dominated by deciduous oak (e.g., Quercus serrata). Three dominant species (Quercus serrata; Fagus crenata; Betula ermanii) were selected for comparison between AFst0 and AOT40. The distributions of the species targeted by this study are shown in Fig. 1. A distribution was determined based on the vegetation data of the National Survey on the Natural Environment, investigated by the Ministry of the Environment (⇒ http://www.biodic.go.jp/J-IBIS.html). Spatial resolution of these data was 1 × 1 km.
Fig. 1 - Distribution of dominant forest species in Japan (A: Quercus serrata; B: Fagus crenata; C: Betula ermanii).
Input data
Climate data (air temperature, air humidity, solar radiation and wind speed) and ozone concentration data for the year 2000 ([33]) were input into our model. These data were estimated using CHASER ([32]) and the WRF/Chem model ([7]) provided at 6h temporal resolution, a spatial resolution of 40 × 40 km across Japan. We used these data as input data near ground surface at 20 m high. Validation for the model estimates of ozone concentration was conducted at several monitoring sites in Japan ([33]). Although further validation and model improvement may be needed, the model estimates were used for estimation of stomatal ozone uptake.
Input data of distributions for deciduous tree species were derived from identifying whether the grid space (40 × 40 km) included the distribution of vegetation data shown in Fig. 1 or not. The estimation of stomatal ozone uptake was conducted in each grid square (40 × 40 km) with 6 h time steps.
Estimation of AOT40
AOT40 was estimated using ozone concentration at the top of the canopy as recommended for ozone risk assessment ([35]). We used ozone concentration data at 20 m high as C (z1). AOT40 was calculated as follows (eqn. 1):
where C (z1)i is averaged ozone concentration for 6 hours at the top of the canopy (ppb), n is the number of data for ozone concentrations. AOT40 was calculated over daylight hours with a solar irradiation higher than 50 W m-2 from April to September (e.g., [13]).
Stomatal conductance model
Leaf-level stomatal conductance of water vapor (gsw) was estimated using the multiplicative model ([10], [3] - eqn. 2):
where g
max is the maximum stomatal conductance. The other functions are limiting factors of g
max and are scaled from 0 to 1. f
min is the minimum stomatal conductance and is set to 0 in this study because we could not get data of g
min from the literatures. f
phen is the variation in stomatal conductance with leaf age, and f
light, f
temp, f
VPD, and f
SMD are functions of photosynthetically photon flux density at the leaf surface (PPFD, μmol photons m-2 s-1), temperature (T, °C), vapor pressure deficit (VPD, kPa), and volumetric soil water content (θ, m3 m-3), respectively.
The variation in stomatal conductance with leaf age (f
phen) modifies g
max as a function of time within the leaf duration ([3]). Maruyama & Honda ([14]) reported that it took about 1 month for g
sw to reach its peak from leaf onset, and to decline due to its senescence until leaf fall for Japanese beech and deciduous oak species. Therefore, we assumed that f
phen increased linearly from 0 to 1 during the first 30 days after leaf onset and decreased linearly from 1 to 0 during the 30 days prior to leaf fall.
The functions (f
light, f
temp and f
VPD) have been expressed in various forms (e.g., [10], [31], [3]). In this study, the following formulas were selected because they have been frequently used in modeling studies in Japan (e.g., [31]). These functions are expressed as follows (eqn. 3, eqn. 4, eqn. 5):
where f
light_0.5 is the value of PPFD when f
light = 0.5, and T
opt, T
min, and T
max represent the optimum, minimum, and maximum temperatures for stomatal conductance, respectively. f
VPD_0.5 is the value of VPD when f
VPD = 0.5, and a
is a constant.
Tab. 1 lists the parameters used in the stomatal conductance model. Parameters for f
light, f
temp, f
VPD, and g
max were determined from a review of scientific literature on stomatal conductance of temperate deciduous forest tree species in Japan: Quercus serrata ([34], [31], [37]), Fagus crenata ([8]), Betula ermanii ([15], [37]). Fig. 2 shows a plot of these functions in the stomatal conductance model.
Tab. 1 - Parameters of the stomatal conductance model for each dominant deciduous tree species in Japan.
Parameter | Deciduous oak (Q. serrata) | Beech (F. crenata) | Birch (B. ermanii) | |||
---|---|---|---|---|---|---|
Value | References | Value | References | Value | References | |
gmax (mmol m-2 s-1) |
480 | Tanaka et al. ([34]), Sirisampan et al. ([31]), Yamazaki et al. ([37]) |
425 | Iio et al. ([8]) | 680 | Muraoka & Koizumi ([15]), Yamazaki et al. ([37]) |
flight_0.5 (μmol m-2 s-1) |
145 | Tanaka et al. ([34]), Sirisampan et al. ([31]) |
90 | Iio et al. ([8]) | 110 | Yamazaki et al. ([37]) |
Topt (°C) | 24 | Tanaka et al. ([34]), Yamazaki et al. ([37]) |
25 | Iio et al. ([8]) | 25 | Yamazaki et al. ([37]) |
Tmin (°C) | 0 | 0 | 0 | |||
Tmax (°C) | 48 | 40 | 45 | |||
FVPD_0.5 (kPa) | 2.1 | Yamazaki et al. ([37]) | 2.4 | Iio et al. ([8]) | 1.2 | Yamazaki et al. ([37]) |
a | 3.4 | 1.9 | 2.5 |
Fig. 2 - Plots of the functions light (A); temp (B); VPD (C) of the stomatal conductance model for three deciduous tree species. light, temp and VPD are functions of photosynthetically photon flux density at the leaf surface (PPFD, μmol photons m-2 s-1), temperature (T, °C), and vapor pressure deficit (VPD, kPa), respectively.
Parameters of f
SMD could not be included in the model because of insufficient published data about f
SMD for temperate deciduous tree species in Japan. The f
SMD values were not calculated in this study (f
SMD=1), although this assumption may lead to an overestimation of stomatal ozone uptake. However, Sirisampan et al. ([31]) reported that the soil water content had no effect on the stomatal conductance for six tree species in central Japan. Therefore, the results would still allow a comparison of the maps between stomatal ozone uptake and AOT40.
Estimation of stomatal ozone uptake
Estimation of stomatal ozone uptake (F
st; nmol O3 m-2 s-1) were calculated based on the assumption that the ozone concentration at the top of the canopy [C (z1)] represented a concentration near sunlit leaves at the top of the canopy ([4]). Then stomatal ozone uptake was calculated as follows (eqn. 6):
where r
b is the leaf boundary layer resistance (s m-1), r
c is the leaf surface resistance [= 1/(g
sw/1.65+g
ext); s m-1], 1.65 accounts for the difference in diffusivity of water in air compared with ozone, and g
ext is the external leaf or cuticular conductance (s m-1). g
ext was set to 0.0004 s m-1 ([4]).
Leaf boundary layer resistance (r
b) was calculated from the wind speed at canopy height, u (z
1) in m s-1, and the mean leaf width, L
d (m, [35] - eqn. 7):
where the factor 1.3 accounts for differences in diffusivity between heat and ozone. L
d for oak and beech/birch were determined as 0.1 and 0.05 m, respectively, from leaf shape data in Japan ([5]).
In Europe, the accumulative stomatal ozone uptake (AF
st Y
) was recommended to assess the ozone risk for forest species ([11]). It is given by the following equation (eqn. 8):
where Y
is a threshold of stomatal ozone uptake (nmol O3 m-2 s-1). In Europe, a threshold Y
is currently used ([4], [11]). However, it is not clear whether the same threshold of F
st can be applied in Japan. Therefore, we did not set a threshold for the F
st value (AFst0) in the present study.
Estimation of leaf duration
Leaf duration was simply assumed based on phenological data in Japan (Tab. 2). We chose the data by including several species in Japan. Because we could not collect enough phenological data for each species, we assumed no difference in leaf duration among species. From these data, a leaf onset time was set depending on the latitude in Japan (leaf onset time = 3.4 · latitude - 9.1; r2 = 0.89). In contrast, time of leaf fall did not show a linear relationship with latitude. Therefore, the averaged value of the time (DOY = 311, s.d. = 17) was adopted as time of leaf fall in this study.
Tab. 2 - Observed time (day of year) of leaf onset and leaf fall for deciduous forest trees in Japan.
Region | Observation (day of year) | References | |
---|---|---|---|
Leaf onset |
Leaf fall |
||
Western Japan (35°10’ N, 132°40’E) | 107 | 339 | Ozaki et al. ([25]) |
Central-eastern Japan (34°55’ N, 137°45’E) | 109 | 319 | Fujimoto ([6]) |
Central-eastern Japan (36°30’ N, 138°20’E) | 121 | 297 | Kato & Hayashi ([12]) |
Northern Japan (39°90’ N ,141°00’E) | 131 | 298 | Aoki & Hashimoto ([1]) |
Hokkaido (42°59’ N, 141°23’E) | 135 | 304 | [19] |
Statistics and data analysis
To compare AOT40 and AFst0, maps of both indices were provided. In addition, averaged AOT40 and AFst0 were calculated for each region. The linear correlations between both indices, and between fVPD in summer and AFst0 were tested. Moreover, difference of the indices among regions was tested with a Kruskal-Wallis test. Results were considered significant at p < 0.05. Statistical analysis was performed with SPSS software version 11.5 (SPSS, Chicago, USA).
Results
Fig. 3 shows a map of the AOT40 values from April to September in Japan, and Tab. 3 shows an averaged value of AOT40 and AFst0 of each region in Japan. There were differences in the AOT40 values among regions. The AOT40 values showed the highest value more than 30 ppm·h from central-eastern Japan (34°-38°N 136°-139°E) to western Japan (31°-35°N 130°-133°E - Fig. 3). In contrast, the AOT40 values were relatively low (0-10 ppm·h) in Hokkaido (42°- 44°N 140°- 145°E).
Tab. 3 - Mean values (± SD) of estimated AOT40 and AFst0 for deciduous tree species in each region of Japan. The values were calculated for each region including more than 5 grids. Within the same parameter, values with different letters are significantly different (Kruskal-Wallis test, p < 0.05 - number of 40 × 40 km grids, p < 0.05).
Region | AOT40 (ppm·h) |
AFst0 (mmol m-2) | ||
---|---|---|---|---|
Quercus serrata | Fagus crenata | Betula ermanii | ||
Hokkaido (42°-44°N, 140°-145°E) | 5.1 ± 1.6 a (41) |
- | 26.7 ± 1.6 a (6) |
31.9 ± 2.3 a (35) |
Eastern Japan (35°-42°N,139°-142°E) | 16.7 ± 5.0 b (53) |
35.9 ± 3.4 a (44) |
35.1 ± 3.2 b (43) |
40.8 ± 4.4 b (14) |
Central-eastern Japan (34°-38°N, 136°-139°E) | 28.0 ± 5.6 c (41) |
41.2 ± 3.7 b (41) |
41.1 ± 2.7 c (32) |
48.3 ± 4.0 c (21) |
Central Japan (33°-36°N, 133°-136°E) | 30.8 ± 3.5 d (31) |
36.0 ± 3.5 a (30) |
40.1 ± 2.1 c (17) |
- |
Western Japan (31°-35°N, 130°-133°E) | 26.4 ± 4.3 c (40) |
40.6 ± 2.3 b (30) |
41.8 ± 2.2 c (19) |
- |
Significance of difference | ||||
Kruskal-Wallis test | < 0.001 | < 0.001 | < 0.001 | < 0.001 |
Fig. 3 - A map of estimated AOT40 values from April to September in Japan along the 40 × 40km grid of the CHASER and WRF/Chem models used to estimate climate and ozone input data.
Fig. 4 (A-C) shows maps of the AFst0 values of the deciduous tree species in Japan. For Quercus serrata, AFst0 showed the highest value in central-eastern Japan (Fig. 4A). The averaged AFst0 values in this region were 41.2 mmol m-2 s-1 (Tab. 3). In this area, the AFst0 values reached about 50 mmol m-2 for Quercus serrata and Fagus crenata, and 55 mmol m-2 for Betula ermanii. In Hokkaido or eastern Japan (35°-42°N 139°-142°E), the AFst0 values were lower than in central-eastern Japan (Fig. 4, Tab. 3). In Hokkaido, the averaged AFst0 values were 26.7 mmol m-2 and 31.9 mmol m-2 for Fagus crenata and Betula ermanii, respectively (Tab. 3). The averaged AFst0 values for Quercus serrata were 35.9 mmol m-2 in eastern Japan (Tab. 3). In central Japan (33°- 36°N 133°-136°E), the AFst0 values were similar to the levels in eastern Japan for Quercus serrata (Fig. 4A), Tab. 3). The AFst0 values showed below 30mmol m-2 for Quercus serrata in this region (Fig. 4A).
Fig. 4 - Maps of estimated AFst0 (accumulated stomatal ozone uptake) values of the deciduous tree species in Japan. (A): Quercus serrata; (B): Fagus crenata; (C): Betula ermanii.
A comparison between the AOT40 and AFst0 maps (Fig. 3, Fig. 4) suggested a difference in the spatial pattern. Although the AOT40 values were different between eastern Japan, and central Japan, the AFst0 values were not significantly different for Quercus serrata (Tab. 3). These areas are the warmer parts of Japan. Fig. 5 shows the averaged fVPD values for Quercus serrata in summer (July-August). In central Japan, the averaged fVPD values were about 0.8 in the summer (Fig. 5) because of high temperature. The lower fVPD values corresponded with the lower AFst0 areas for Quercus serrata.
Fig. 5 - Spatial distribution of averaged fVPD values for Quercus serrata from July to August in Japan. fVPD shows the response of stomatal conductance to vapor pressure deficit, scaled from 0 to 1.
For further analysis, we conducted a correlation analysis between both indices in Japan (Tab. 4). The result shows that the correlation between AOT40 and AFst0 was lower for Quercus serrata compared to other two species. Quercus serrata is mainly distributed in warm-temperate climate region (Fig. 1). Especially, the correlation was not significant in warmer part of Japan such as central and western Japan (Tab. 4). This result was influenced by effects of VPD on stomatal conductance (Fig. 5, Tab. 5).
Tab. 4 - Correlation between AOT40 and AFst0 (accumulative stomatal ozone uptake) for each tree species in Japan. The correlation coefficient was calculated for each area including more than 5 grids. (*) and (**) denotes the significance at 5% and 1 % level, respectively. (NS) indicates no significant correlation.
Region | Quercus serrata | Fagus crenata | Betula ermanii |
---|---|---|---|
All regions | 0.33** | 0.89** | 0.91** |
Hokkaido - Central-eastern Japan (34°-44°N, 136°-145°E) | 0.53** | 0.93** | 0.93** |
Central Japan - Western Japan (31°-36°N, 130°-136°E) | -0.06 NS | 0.34* | - |
Tab. 5 - Correlation between fVPD (response of stomatal conductance to vapor pressure deficit) in summer and AFst0 (accumulative stomatal ozone uptake) for Quercus serrata in Japan. The correlation coefficient was calculated for each area including more than 5 grids. (*) and (**) denotes the significance at 5% and 1 % level, respectively. (NS) indicates no significant correlation.
Region | Value |
---|---|
All regions | 0.43** |
Hokkaido - Central-eastern Japan (34°-44°N, 136°-145°E) | 0.38** |
Central Japan - Western Japan (31°-36°N, 130°-136°E) | 0.9** |
For the other two species distributed in humid and cool region and high mountainous area, the correlation between AOT40 and AFst0 was very high (Tab. 4). Fagus crenata is also distributed in the warmer part of Japan such as central and western Japan (Fig. 1). In the warmer part of Japan, the correlation between AOT40 and AFst0 decreased for Fagus crenata (r = 0.37).
Discussion
The purpose of this study was to compare the spatial map of the AFst0 and AOT40 values for deciduous forest trees in Japan. Emberson et al. ([3]) and Simpson et al. ([30]) similarly compared the spatial distribution of the stomatal ozone uptake and AOT40 values in Europe. The AOT40 value was relatively high in southern Europe compared to northern Europe. The spatial gradient of the AOT40 values was remarkable from south to north, but the spatial gradient of the stomatal ozone uptake was much less. Stomatal ozone uptake was limited due to drought stress during the summer in Mediterranean regions where ozone concentrations were high ([26]).
In this study, AFst0 showed similarly much less regional differences than AOT40. However, there is a difference of the result compared to Europe. In Europe, the exposure-based critical level for forest species is suggested. Values of the critical levels are defined as 5 ppm·h of AOT40. AOT40 values exceeding the European critical levels were shown in 89% of Japan. In Japan, Kohno et al. ([13]) suggested that 15-30 ppm·h AOT40 for moderately sensitive species (e.g., Quercus serrata) from April to September as critical level for forest species. AOT40 values exceeding 30 ppm·h were shown in 21% of Japan. Some regions where the AOT40 values reached 30 ppm·h corresponded to cool and humid climate such as central-eastern Japan. Averaged stomatal ozone uptake was estimated to be higher than 40 mmol m-2 for the three species in central-eastern Japan (Tab. 3). In contrast, stomatal ozone uptake for Quercus serrata did not show any difference between eastern and central Japan, although AOT40 in central Japan was twice that in eastern Japan. Emberson et al. ([3]) showed that VPD played a major role in limiting the stomatal ozone uptake. Also in this study, VPD is a limiting factor of the stomatal ozone uptake especially in warmer part of Japan (Tab. 5). For this reason, this region showed a discrepancy between the AFst0 values of Quercus serrata and AOT40. These results suggest that not only ozone concentration but also stomatal closure induced by VPD affected the AFst0 in the warmer part of Japan.
The results of correlation analysis for Fagus crenata and Betula ermanii suggested that AFst0 and AOT40 values are similar for ozone risk assessment in humid and cool climate region, because the correlation between AOT40 and AFst0 was very high (Tab. 4), i.e., humid and cool climate was favorable for stomatal opening. However, in the warmer part of Japan, the correlation between AOT40 and AFst0 decreased for Fagus crenata (r = 0.37), because VPD induced a stomatal closure.
In warmer part of Japan, we also could find the difference of spatial pattern of the AFst0 values between Fagus crenata and Quercus serrata (Fig. 4). Stomatal conductance for Fagus crenata was less sensitive to high VPD (>2 kPa) than that for Quercus serrata (Tab. 1; Fig. 2). Therefore, the result is due to the species-specific characteristics of the stomatal conductance parameters. The assessment approach using AOT40 does not consider the difference of species-specific stomatal response to the climate factors. The result of this study illustrated the limitations of performing ozone risk assessment using AOT40 in Japan.
In this study, f
SMD could not be applied in the model because of insufficient published data about soil moisture deficit. However, Sirisampan et al. ([31]) reported that the soil water content had no effect on the stomatal conductance of six tree species in central Japan. However, soil water deficit may be a limiting factor in estimating AFst0 even for short periods during a growing season. The parameter of f
SMD would bring about a further refinement of the calculations for Japan. In addition, we assumed that plants absorbed ozone continuously when climate condition was favorable for stomatal opening. However, when leaves experience a longer period of ozone exposure, stomatal response would be changed ([27]). Pleijel et al. ([29]) and Danielsson et al. ([2]) reported that ozone exposure over a long time caused a decline in g
max for crops in their modelling studies. This effect has not included in the model of this study yet. Long-term ozone exposure would also be limiting factor of stomatal ozone uptake. Including the effects of ozone on stomatal conductance would be expected to improve the ozone uptake modelling for deciduous forests in Japan.
Conclusions
The maps between AFst0 and AOT40 for dominant temperate deciduous tree species in Japan were compared. Estimations of stomatal ozone uptake were accomplished using estimated ozone concentration and climate data, and vegetation data. As a result, stomatal closure induced by vapour pressure deficit affected the AFst0 values in warmer parts of Japan.
Therefore, the areas with the highest AOT40 did not correspond to the areas with the highest AFst0, suggesting that the use of AOT40 for protecting Japanese forests may be misleading.
Stomatal ozone uptake was suggested for assessing ozone damage more than three decades ago in Japan ([22]). Many studies (e.g., [28]) suggested that the stomatal flux-based approach is scientifically-sound and would be a useful tool for ozone risk assessment. This paper is a contribution to develop the stomatal flux-based approach in Japan. Future work should validate and improve our model with field measurements. Parameterizations for more species would also contribute further developments because ozone sensitivity of plants and stomatal response to environmental condition are species-specific (e.g., [23]).
Acknowledgements
This work was supported by JSPS Fellowships for Young Scientists and was partly supported by the Global Environment Research Fund (C-062) of the Ministry of the Environment, Japan.
References
Gscholar
Gscholar
Gscholar
Gscholar
Gscholar
Gscholar
Gscholar
Gscholar
Gscholar
Gscholar
Gscholar
Gscholar
Gscholar
Authors’ Info
Authors’ Affiliation
Corresponding author
Paper Info
Citation
Hoshika Y, Shimizu Y, Omasa K (2011). A comparison between stomatal ozone uptake and AOT40 of deciduous trees in Japan. iForest 4: 128-135. - doi: 10.3832/ifor0573-004
Paper history
Received: Feb 15, 2011
Accepted: Mar 31, 2011
First online: Jun 01, 2011
Publication Date: Jun 01, 2011
Publication Time: 2.07 months
Copyright Information
© SISEF - The Italian Society of Silviculture and Forest Ecology 2011
Open Access
This article is distributed under the terms of the Creative Commons Attribution-Non Commercial 4.0 International (https://creativecommons.org/licenses/by-nc/4.0/), which permits unrestricted use, distribution, and reproduction in any medium, provided you give appropriate credit to the original author(s) and the source, provide a link to the Creative Commons license, and indicate if changes were made.
Web Metrics
Breakdown by View Type
Article Usage
Total Article Views: 32433
(from publication date up to now)
Breakdown by View Type
HTML Page Views: 26520
Abstract Page Views: 1454
PDF Downloads: 3239
Citation/Reference Downloads: 53
XML Downloads: 1167
Web Metrics
Days since publication: 4805
Overall contacts: 32433
Avg. contacts per week: 47.25
Article Citations
Article citations are based on data periodically collected from the Clarivate Web of Science web site
(last update: Nov 2020)
Total number of cites (since 2011): 16
Average cites per year: 1.60
Publication Metrics
by Dimensions ©
Articles citing this article
List of the papers citing this article based on CrossRef Cited-by.
Related Contents
iForest Similar Articles
Research Articles
Prediction of ozone effects on net ecosystem production of Norway spruce forest
vol. 11, pp. 743-750 (online: 15 November 2018)
Research Articles
Soil drench of ethylenediurea (EDU) protects sensitive trees from ozone injury
vol. 4, pp. 66-68 (online: 05 April 2011)
Research Articles
Effects of abiotic stress on gene transcription in European beech: ozone affects ethylene biosynthesis in saplings of Fagus sylvatica L.
vol. 2, pp. 114-118 (online: 10 June 2009)
Research Articles
Changes in the proteome of juvenile European beech following three years exposure to free-air elevated ozone
vol. 4, pp. 69-76 (online: 05 April 2011)
Short Communications
Ozone flux modelling for risk assessment: status and research needs
vol. 2, pp. 34-37 (online: 21 January 2009)
Research Articles
A new approach to ozone plant fumigation: The Web-O3-Fumigation. Isoprene response to a gradient of ozone stress in leaves of Quercus pubescens
vol. 1, pp. 22-26 (online: 28 February 2008)
Research Articles
Ozone fumigation effects on the morphology and biomass of Norway spruce (Picea abies L.) saplings
vol. 2, pp. 15-18 (online: 21 January 2009)
Research Articles
Ambient ozone phytotoxic potential over the Czech forests as assessed by AOT40
vol. 5, pp. 153-162 (online: 25 June 2012)
Research Articles
Photosynthesis of three evergreen broad-leaved tree species, Castanopsis sieboldii, Quercus glauca, and Q. myrsinaefolia, under elevated ozone
vol. 11, pp. 360-366 (online: 04 May 2018)
Short Communications
QA/QC activities and ecological monitoring in the Acid Deposition Monitoring Network in East Asia (EANET)
vol. 2, pp. 26-29 (online: 21 January 2009)
iForest Database Search
Search By Author
Search By Keyword
Google Scholar Search
Citing Articles
Search By Author
Search By Keywords
PubMed Search
Search By Author
Search By Keyword