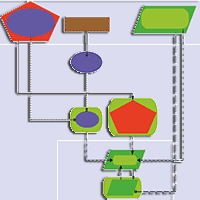
The impact of land use on future water balance - A simple approach for analysing climate change effects
András Herceg , Péter Kalicz, Zoltán Gribovszki
iForest - Biogeosciences and Forestry, Volume 14, Issue 2, Pages 175-185 (2021)
doi: https://doi.org/10.3832/ifor3540-014
Published: Apr 13, 2021 - Copyright © 2021 SISEF
Research Articles
Abstract
Regional climate change projections for Europe agree in predicting a statistically significant warming in all seasons. The most significant climate change effect is its impact on water cycle through altering precipitation patterns and evapotranspiration processes at multiple scales. The anticipated changes in the distribution and precipitation amounts together with continuously increasing temperatures may induce a higher rate of water consumption in plants, which can generate changes in soil moisture, groundwater, and the water cycle. Thus, climate change can cause changes in the water balance equations structure. A Thornthwaite-type monthly step water balance model was established to compare the water balance in three different surface land cover types: (i) a natural forested area; (ii) a parcel with mixed surface cover; (iii) an agricultural area. The key parameter of the model is the water storage capacity of the soil. Maximal rooting depth of the given area is also determinable during the calibration process using actual evapotranspiration (AET) and soil physical data. The locally calibrated model was employed for assessing future AET and soil moisture of selected land cover types using data from four bias-corrected regional climate models. The projections demonstrate increasing actual evapotranspiration values in each surface cover type at the end of the 21st century. Regarding the 10th percentile minimum soil moisture values, the forested area displayed an increasing trend, while the agricultural field and mixed parcel showed a strong decrease. The 30-year monthly means of evapotranspiration shows the maximum values in June and July, while the minimum soil moisture in September. Water stress analysis indicates water stress is expected to occur only in the agricultural field during the 21st century. The comparison of the three surface covers reveals that forest has the greatest soil water storage capacity due to the highest rooting depth. Thus, according to the projections for 21st century, less water stress is predicted to occur at the forested area compared to the other two surface covers which shows shallow rooting depth.
Keywords
Water Balance, Climate Change, Plant Available Water, Evapotranspiration, Soil Moisture, Water Stress
Authors’ Info
Authors’ address
Péter Kalicz 0000-0003-0010-9519
Zoltán Gribovszki 0000-0003-3061-8912
Institute of Geomatics and Civil Engineering, University of Sopron, Sopron (Hungary)
Corresponding author
Paper Info
Citation
Herceg A, Kalicz P, Gribovszki Z (2021). The impact of land use on future water balance - A simple approach for analysing climate change effects. iForest 14: 175-185. - doi: 10.3832/ifor3540-014
Academic Editor
Raffaele Lafortezza
Paper history
Received: Jun 01, 2020
Accepted: Feb 05, 2021
First online: Apr 13, 2021
Publication Date: Apr 30, 2021
Publication Time: 2.23 months
Copyright Information
© SISEF - The Italian Society of Silviculture and Forest Ecology 2021
Open Access
This article is distributed under the terms of the Creative Commons Attribution-Non Commercial 4.0 International (https://creativecommons.org/licenses/by-nc/4.0/), which permits unrestricted use, distribution, and reproduction in any medium, provided you give appropriate credit to the original author(s) and the source, provide a link to the Creative Commons license, and indicate if changes were made.
Web Metrics
Breakdown by View Type
Article Usage
Total Article Views: 5166
(from publication date up to now)
Breakdown by View Type
HTML Page Views: 2819
Abstract Page Views: 350
PDF Downloads: 1584
Citation/Reference Downloads: 2
XML Downloads: 411
Web Metrics
Days since publication: 1201
Overall contacts: 5166
Avg. contacts per week: 30.11
Citation Metrics
Article Citations
Article citations are based on data periodically collected from the Clarivate Web of Science web site
(last update: Nov 2020)
(No citations were found up to date. Please come back later)
Publication Metrics
by Dimensions ©
Articles citing this article
List of the papers citing this article based on CrossRef Cited-by.
References
Allgemeine Hydrologie, Quantitative Hydrologie, In: “Lehrbuche der Hydrologie, Band 1” [General Hydrology, Quantitative Hydrology, In: “Textbooks of Hydrology”, vol. 1]. Berlin, Stuttgart, Germany, pp. 320-350. [in German]
Gscholar
Physical hydrology (2nd edn). Prentice Hall, Upper Saddle River, NJ, USA, pp. 646.
Gscholar
Creation of the FORESEE database to support climate change related impact studies, In: Proceedings of the “International Scientific Conference for PhD Students”. Györ (Hungary) 19-20 Mar 2013. University of West Hungary Press, Sopron, Hungary, pp. 1-5.
Gscholar
Magyarország kistájainak katasztere - második, átdolgozott és bövített kiadás [Inventory of microregions in Hungary]. Hungarian Academy of Sciences, Geographical Institute, Budapest, Hungary, pp 299, pp. 345-347. [in Hungarian]
Gscholar
Estimation of water stress by comparing the Thornthwaite water balance model with a tree ring width analysis. In: Proceedings of the Conference “HydroCarpath 2015, Catchment Processes in Regional Hydrology”. Nyugat-magyarországi Egyetem Kiadó, Sopron (Hungary) 29 Oct 2016, pp. 6.
Online | Gscholar
BCRRA Predicting subgrade soil strength using FWD and meteorological time series data. In: Proceedings of the “10th International Conference on the Bearing Capacity of Roads, Railways and Airfields (BCRRA 2017)” (Loizos A, Al-Qadi I, Scarpas T eds). Athens (Greece) 28-30 June 2017. Taylor & Francis Group, London, UK, pp. 2117-2125.
Gscholar
Biologische Landwirtschaft im Marchfeld. Potenziale zur Entlastung des Natur- und Landschaftshaushaltes [Organic farming in Marchfeld. Potential for relieving the natural and landscape balance]. Umweltbundesamt GmbH, Vienna, pp. 34-36. [in German]
Gscholar
Technical Summary, 2019. In: “Climate Change and Land. An IPCC Special Report on climate change, desertification, land degradation, sustainable land management, food security, and greenhouse gas fluxes in terrestrial ecosystems” (Shukla PR, Skea J, Calvo Buendia E, Masson-Delmotte V, Pörtner H-O, Roberts DC, Zhai P, Slade R, Connors S, van Diemen R, Ferrat M, Haughey E, Luz S, Neogi S, Pathak M, Petzold J, Portugal Pereira J, Vyas P, Huntley E, Kissick K, Belkacemi M, Malley J. eds). IPCC, Japan, pp. 37-74.
Online | Gscholar
Klímaváltozás hatásának vizsgálata a Bácsbokodi-Kígyós csatorna vízgyüjtöjén Budyko-modell alkalmazásával [Investigation of the impact of climate change in the catchment of the Bácsbokodi-Kígyós canal using the Budyko model]. In: “A Magyar Hidrológiai Társaság XXVIII, Országos Vándorgyülése”. Sopron (Hungary) 7-9 Jul 2010. Magyar Hidrológiai Társaság, Budapest, pp. 1207-1225. [in Hungarian]
Gscholar
Sopron Környéki Üledékek Összefoglaló Földtani Értékelése [Geological assessement of sediments in the neighbourhood of Sopron]. Manuscript, Sopron, Hungary, pp. 48. [in Hungarian]
Gscholar
Tó- és területi párolgás becslésének pontosítása és magyarországi alkalmazásai [Specifying lake and areal evapotranspiration rates in Hungary]. PhD thesis, Vásárhelyi Doctoral School of Civil Engineering and Earth Sciences, Budapest University of Technology and Economics, Budapest, Hungary, pp. 92-94. [in Hungarian]
Gscholar
Forest hydrology: an introduction to water and forests (2nd edn). Austin State University, Austin, TX, USA, , pp. 181.
Gscholar
Segmented: an R package to fit regression models with broken-line relationships. R News, vol. 8/1, pp. 20-25.
Gscholar
Influence of climate change on the hydrosphere. In: “Austrian Assessment Report Climate Change 2014 (AAR14)”. Austrian Panel on Climate Change (APCC), Austrian Academy of Sciences Press, Vienna, Austria, pp. 411-466.
Gscholar
Modeling potential evapotranspiration of two forested watersheds in the southern Appalachians. Soil and Water Division of ASABE, American Society of Agricultural and Biological Engineers, vol 54 (6): 2067-2078.
Gscholar
Effects of climate change on the hydrological cycle in Central and Eastern Europe. In: “Managing Protected Areas in Central and Eastern Europe under Climate Change” (Rannow S, Neubert M eds). Springer, Dordrecht, Netherlands, pp. 31-43.
CrossRef | Gscholar
A calibration-free evapotranspiration mapping (CREMAP) technique. In: “Evapotranspiration” (Labedzki L ed). INTECH, Rijeka, Croatia, pp. 257-274.
Gscholar
The water balance. Climatological Laboratory Publication no. 8, Drexel Institute of Technology, Philadelphia, PA, USA, pp. 104.
Gscholar
ENSEMBLES: climate change and its impacts: summary of research and results from the ENSEMBLES project. Met Office, Hadley Centre, Exeter, UK, pp. 160.
Gscholar